Аннотация
Animal food-borne microbes are pathogens that jeopardize food safety and cause illness in humans via natural infection or contamination. Most of those microbes are bacteria that have considerable impacts on public health. Their survival and pathogenicity are due to toxin production, biofilm development, spore formation, disinfection resistance, and other traits. However, detailed information about them is scattered across scientific literature.We aimed to compile information about major zoonotic bacteria linked with human food of livestock origin and describe their typical features, transmission modes, detection, and preventative approaches. In particular, we addressed the following pathogens that cause food-borne disease worldwide: Campylobacter, Salmonella, Listeria, Staphylococcus, Brucella, Clostridium, Mycobacterium, Colibacilus, and some others.
Many of those bacteria have substantial reservoirs in food animals, and food products of animal origin are the primary vehicles of their transmission. Human beings become affected by food-borne zoonotic bacteria if they consume raw animal products or foods produced by using unstandardized slaughtering methods or unsanitary preparation and handling procedures. These zoonotic bacteria and their toxins can be detected in food by culturing, serological, and molecular diagnostic methods. They are effectively controlled and prevented by good hygiene, good management practices, cooking, and pasteurization protocols.
In addition, there is a need for a centralized surveillance and monitoring system, as well as higher awareness in society of the occurrence, prevention, and control of bacterial pathogens related to food animals.
Ключевые слова
Bacteria, characteristics, control, food-borne disease, livestock, pathogens, prevention and transmissionsВВЕДЕНИЕ
Human beings significantly benefit from livestock products and services. Livestock occupies around 22– 26% of ice-free land of the globe, with feed production covering one-third of farmland [1]. Livestock production accounts for almost 40% of the global agricultural GDP in Africa, as well as 33% of protein and 17% of all calories consumed worldwide [2]. The production also provides significant employment opportunities for rural residents [3]. Furthermore, cattle are an important source of food, nutrition, livelihood, and income in underdeveloped countries [4]. It provides industrial raw materials (milk, meat, hides, and skin), as well as high-value protein, to Ethiopian consumers [5].
Foods of livestock origin play an important role in human diet. Nutritionally, they are an important source of protein, carbohydrates, vitamins, minerals, and other nutrients of good quality. In addition, animal foods are generally more distinctive in flavor or texture and are often more palatable than foods of vegetable origin. However, these livestock-originated foods can be contaminated at any point in the food supply chain: during production, processing, distribution, preparation, and/or final consumption [6]. This results in food-borne disease transmitted to humans through direct or indirect contact with the animal source or related goods. The consumption of infected raw livestock products, such as milk, meat, and eggs, is a major way of transmission [7].
Food-borne diseases, especially those associated with livestock products, are a global public health threat [8]. The occurrence of food-borne diseases in humans increases as they consume more animal products [9]. The increased consumption of animal products (meat, milk, and eggs) is fueled by a rapid human population growth, urbanization, rising per capita income, globalization, and changing consumer preferences. This leads to a large-scale manufacturing and global transportation of livestock products with exposure to contaminants and food-borne disease transmission [10].
Microorganisms and their toxins, as well as natural toxins, adulterants, and other probable contaminants in animal products (meat, milk, egg, fish, etc.) pose a substantial risk to people [10]. This risk is mostly due to poor farming, slaughtering, processing, and cleaning techniques used. Moreover, a lack of veterinary services and insufficient food safety regulations are common causes of food-borne diseases in developing countries, including Ethiopia [11, 12].
Bacterial pathogens associated with foods originating from animals and resulting in food-borne diseases in humans include Salmonella, Campylobacter, Escherichia, Listeria, Staphylococcus, Brucella, Clostridia, Mycobacteria, and others [13]. They are the main source of food spoilage and food-borne infections [6]. Food-borne bacteria are a huge threat to public health, and they can cause illness in humans when they consume animal products contaminated with germs or their toxins [12].
Studies conducted in many parts of the world have demonstrated that certain bacterial infections related to animal-derived foods pose a major threat to public health [10]. The majority of their findings, however, are scattered across different articles. Yet, scientific communities and other stakeholders need structured information on this subject.
Therefore, we aimed to:
– review major zoonotic bacterial pathogens associated with foods of livestock origin;
– highlight typical features, detection methods, and transmission modes of these pathogens, as well as prevention and control measures to overcome their effect on humans.
РЕЗУЛЬТАТЫ И ИХ ОБСУЖДЕНИЕ
Zoonotic bacteria of livestock-originated food. Bacterial pathogens are the most serious concern among biological risks in terms of food safety for consumers, and they are among the most common global public health problems in recent times [14, 15]. Gram-negative bacteria are responsible for about 69% of all cases of bacterial food poisoning [16].
Even though a variety of pathogens have been identified as causing food-borne diseases, bacteria, such as Staphylococcus, Salmonella, Campylobacter, Listeria, Escherichia, Brucella, Clostridium, and Mycobacterium, are the most common causes of food-borne disease in humans due to the consumption of animal-originated foods [6, 13].
Campylobacter. Characteristics. Campylobacter belongs to the Campylobacteria family which contains the Arcobacter and Bacteroides genera. Gram-negative, curved or spiral, and microaerophilic bacteria make up the Campylobacter genus. This bacterium has 28 species and 8 subspecies. Thermophilic Campylobacter species, particularly Campylobacter jejuni and Campylobacter coli, are significant food-borne pathogens of livestock products among these species. The most commonly reported Campylobacter species is C. jejuni, followed by C. coli [12].
Some Campylobacter species are thermotolerant and can survive at temperatures ranging from 37 to 42°C, with 42°C and pH 6.5–7.5 being ideal for C. coli and C. jejuni. They are ubiquitous in nature and have been observed colonizing gastrointestinal tracts of wild and domesticated birds and mammals, as well as all food animals [11].
Source and mode of transmission. Campylobacter is found in 100% of poultry (including chickens, turkeys, and waterfowl), cattle, sheep, pigs, and other food animals, as well as wild animals and birds [15]. Human exposure to this pathogen occurs mainly from the consumption of contaminated meat (beef, pork, etc.), unpasteurized milk, and milk products like cheese, as well as from handling and preparation of contaminated foods of livestock origin [11, 17, 18].
As we can see in Fig. 1, Campylobacter invades the gastrointestinal tract of animals such as cattle and poultry. Due to the spillage of Campylobacter-rich intestinal material, the surface contamination of carcasses is high at the slaughterhouse. Therefore, undercooked meat from these animals poses a threat of pathogen transmission to human after ingestion [19].
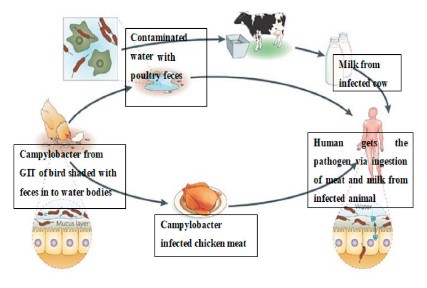
The consumption of unpasteurized milk is one of the main routes of C. jejuni transmission to people in both developed and developing countries [21]. The partial failure of milk pasteurization in the United Kingdom causes C. jejuni to spread from cattle to people [22]. Unpasteurized raw milk has been linked to 80% of C. jejuni infection occurrences in California. Contact with cow feces or polluted water, as well as direct contamination due to bovine mastitis, are all potential sources of milk contamination. Pigs are frequently infected with C. jejuni and C. coli, which should not be underestimated. According to a study conducted in the United States, contaminated meat, pork, and game were responsible for 5% of Campylobacteriosis outbreaks between 1997 and 2008 [23].
Detection. Selective culture media, immunological techniques, and molecular approaches have been used to identify Campylobacter. Selective agars, such as Preston, Charcoal-cefoperazone-deoxycholate, and Butzler agars, have been developed and tested for their effectiveness in isolating Campylobacter. Food samples can be cultured directly onto a selective media. Following isolation, Campylobacter bacteria identification is carried out based on their morphological, biochemical, and growth characteristics [22].
Biochemical assays, such as the enzyme-linked immunosorbent assay (ELISA), can be employed as an alternative to growth on agar, as well as to biochemical or phenotypic identification of Campylobacter. The polymerase chain reaction (PCR) has recently gained popularity for molecular detection and identification of Campylobacter [24].Prevention and control. The majority of Campylobacter illnesses comes from eating infected foods of animal origin such as meat, milk, and eggs. Therefore, Campylobacter infections could be prevented by avoiding the consumption of unpasteurized milk or undercooked meat. To minimize bacterial populations in animals, sanitation and hygiene in livestock barns are essential [25]. Campylobacter is found in domestic and wild animals, as well as in the environment. Controlling Campylobacter infections in humans should start with measures to minimize infection rates in animal reservoirs utilized for food production, and therefore the risk of their presence in animal-sourced food. Farms should use hygiene barriers to keep out wild animals, perform regular check-ups of animals, and improve their handwashing facilities [26].
Campylobacter-positive animals are separated and positive poultry flocks are slaughtered to minimize the spread of contamination. Disinfection of feed and water equipment, as well as proper treatment of their dung, has also been suggested to minimize the incidence of Campylobacter colonization in cattle [25].
The digestive tract of animals contains a large number of Campylobacter species, a possible pollutant of slaughterhouses, environment, and food items. The risk of infection during animal food processing can be minimized by organic acid treatment, UV light, and chemical dip tanks for carcasses. Destroying pathogens or limiting cross-contamination in the kitchen via washing, freezing, or irradiating can help reduce the bacterial transmission to human [27].
Hand washing and separation of ready-to-eat and raw foods are recommended. Cutting boards and utensils that have been used to handle raw meats should be rinsed in hot soapy water before being used to prepare other raw foods. Anyone suffering from an acute diarrheal sickness should avoid food preparation areas until their condition improves. Raw meat, unpasteurized dairy products, and exposure to animals with diarrhea should also be avoided. Before eating, everyone should wash their hands, especially those who work with animals [27, 28].
Non-typhoidal Salmonella. Salmonella infection in humans results in one of two outcomes: self-limiting gastroenteritis or invasive systemic typhoid fever which is mostly caused by the infecting serovars. Salmonella serovars are classified into typhoidal and non-typhoidal Salmonella. Salmonella enteritidis and Salmonella typhi- murium are the most frequent non-typhoidal serovars that cause gastroenteritis. Salmonella typhi and Salmonella paratyphi are typhoidal serovars that cause typhoid fever. About 10–20 million cases of typhoid occur each year, resulting in 100 000–200 000 fatalities in humans worldwide [29, 30].
S. enterica is extremely diverse, with over 2600 distinct serovars. This necessitates understanding the differences between typhoidal and non-typhoidal Salmonella as both are of the same species but cause different disease manifestations. Compared to typhoidal strains that are human host-specific, S. typhimurium and S. enteritidis have broad host ranges. In terms of epidemiology, non-typhoidal Salmonella affects people all over the world, whereas typhoidal Salmonella is mostly found in underdeveloped nations, such as Southeast Asia and Africa. This could be due to a contaminated living environment and low living standards [31].
Characteristic. Salmonella strains are Gram-negative, non-spore-forming, rod-shaped, and motile bacteria that belong to the Enterobacteriaceae family [32]. Salmonella multiplies best at 35–37°C, pH 6.5–7.5, with little or no oxygen [33]. These heat-sensitive bacteria cannot withstand temperatures > 70°C but they can survive in dried feces, dust, and other dry materials, such as feed and some meals, for a long time [34]. The most common serovars of Salmonella that infect livestock and human are shown in Table 1.

Source and mode of transmission. Intestinal tracts of animals (agricultural animals, birds, and reptiles) and people are the principal habitat of Salmonella species. Human food-borne illnesses are caused by Salmonella transmission from animal products [35]. Human salmonellosis is usually caused by the ingestion of contaminated animal products such as meat, pork, and milk [33].
Infected animals used in food production or contaminated carcasses and edible organs are the most common causes of human illness [36]. During slaughtering operations, intestinal content can cross-contaminate carcasses with S. typhimurium DT104, a multidrug-resistant definitive type that is primarily transmitted through the consumption of infected beef. Meat usually contains several of the serovars linked to human illnesses. S. typhimurium, S. enteritidis, and Salmonella heidelberg were three of the most common serotypes detected in meat over the last decade [11].
In milk, contamination can arise through a variety of routes. During the febrile stage of the disease, animals may excrete the organisms in milk. Contaminated feces from either a clinically diseased cow or a healthy carrier may contaminate milk during the milking process. Milk can also be contaminated by dairy workers who use unclean water from dirty equipment. Salmonella-infected food handlers or sewage-polluted water can contaminate food directly or indirectly. The contaminated milk, in its turn, results in human illness if consumed before proper pasteurization [37].
Since pork is the third most often contaminated meat after fresh chicken and turkey, it is the leading cause of human salmonellosis in the EU. Therefore, monitoring and surveillance efforts have been undertaken across the food chain to assess the danger posed by pork to the general population and to avoid Salmonella-borne outbreaks. Pigs can become infected with Salmonella from a contaminated environment, feed, or direct contact with diseased animals. At the slaughterhouse, carcasses can be cross-contaminated with excrements of infected or carrier animals causing a public health risk if consumed raw [38].
Detection. Salmonella surveillance and monitoring should be based on accurate and efficient detection technologies to ensure food safety. New selective media, modified or adapted conventional processes, immunology-based assays, and nucleic acid-based tests are among commercially available, quick methods for Salmonella identification [39]. Selective enrichment media, such as Salmonella Shigella agar, Hekaton enteric agar, or deoxycholate agar, as well as broth, are used to culture and isolate bacteria. Food-borne infections are identified with immunology-based approaches such as ELISA, latex agglutination tests, and immunodiffusion tests [40]. Salmonella pathogens are detected via PCR, which is a molecular assay [399].
Prevention and control. To control and prevent food spoilage caused by Salmonella, biosecurity practices must be used in addition to improved food processing methods, as well as preparation and storage practices [15]. Salmonellosis can be reduced by using attenuated DNA recombinant live Salmonella vaccines in combination with a comprehensive control plan in animals and feeds. Humans, especially vulnerable groups, should avoid eating raw or uncooked meat, drinking raw milk or unpasteurized dairy products, as well as cross-contaminating raw and ready-to-eat foods. After coming into contact with animal feces, people should wash their hands properly [35].
Food processing and preparation should involve cooking, reheating, pasteurization of milk, sufficient refrigeration, and the removal of pets and other animals from food-handling areas [14]. After handling uncooked food, cutting boards, knives, and other tools should be washed thoroughly. Uncooked meat should be stored away from ready-to-eat cooked food. Additional secondary contamination control techniques could include cleaning and disinfection, personnel hygiene, and adequate processing [35].
Listeria. Characteristics. Gram-positive, motile, facultatively anaerobic, non-spore producing, and rod-shaped bacteria make up the Listeria genus [41, 42]. Of ten species in the genus, Listeria monocytogenes is the only one that causes listeriosis in humans. The bacterium can survive at cold temperatures, low pH, and high salt concentrations [11]. In a study by Shamloo et al., L. monocytogenes survived a salt concentration of 21% at 4°C and pH = 4 for 19 days [43]. The bacterium is also resistant to disinfectants and can adhere to a variety of surfaces. L. monocytogenes can generate biofilm, which can be a cause of contamination in the food processing industry [44].
Source and mode of transmission. Raw foods originating from livestock are the most common sources of Listeria species [45]. Foods containing L. monocytogenes include ready-to-eat meat products, ground beef, fish and fish products, milk, and pasteurized dairy products such as soft cheese and ice cream [41, 46].
Farmers, butchers, poultry workers, and veterinary surgeons have been reported to contract listeriosis from animal sources as an occupational hazard [46]. At risk for invasive listeriosis are immunocompromised hosts, such as pregnant women, unborn or newly delivered newborns, organ transplant recipients, cancer and acquired immune deficiency syndrome (AIDS) patients, as well as the elderly [46].
In humans, the most prevalent route of infection is through eating raw animal products such as meat, milk, and pork [47]. The transmission of listeria to humans is also facilitated by cross-contamination of ready-to-eat foods with raw animal products. The highest incidence of L. monocytogenes was found in cow meat (26%) followed by goat meat (25%), and sheep meat (17.9%), indicating that red meat is a vehicle for transmission of L. monocytogenes to humans [45].
Due to low silage quality and insufficient hygiene, the dairy farm is a favorable environment for the survival and spread of L. monocytogenes from cattle into the food chain. L. monocytogenes survival in dairy products varies depending on the kind of product. For example, due to reduced pH and moisture content, pathogen development is nearly impossible in hard cheese when compared to soft or semi-soft cheeses. The first listeriosis outbreak in Europe was linked to fresh cheese, and according to an EU baseline study, 0.47% of retail cheese samples tested positive for L. monocytogenes [48].
In pork, L. monocytogenes is usually discovered in comminuted meats and is found less frequently in freshly-slaughtered pig tissues than in minced pork. Contamination can also arise through the use of tools, hands, boots, gloves, and aprons by manufacturing staff [49].
Detection. Among the procedures used to detect L. monocytogenes is enrichment in selective media, subsequent plating on agar plates, and other tests for species identification. Frequently employed is the two-stage enrichment approach with isolation on polymyxin acriflavine lithium-chloride Ceftazidime aesculin mannitol (PALCAM) agar and Oxford agar [50]. Molecular approaches are also increasingly being utilized to identify L. monocytogenes from food since they are accurate, sensitive, and specific [41].
Prevention and control. Listeriosis can be avoided by thoroughly cleaning all food contact surfaces [51]. To prevent listeriosis and establish consistent management strategies, food-safety control measures must be correctly applied. The most acceptable operational approaches are good hygiene, good manufacturing practice, and sanitation. To limit the risk of infection, vulnerable people (pregnant women, the elderly, and those with compromised immune systems) should avoid unpasteurized dairy products [52]. Food should be protected against L. monocytogenes infection by standardized regulatory rules and quality monitoring of meat products. Preventing the spread of bacteria at different stages of the food production chain is one of the most significant techniques to safeguard food from harmful microbes [41].
In the meat industry, non-food contact surfaces, particularly floors and drains, can be a reservoir of L. monocytogenes [49]. These areas must be cleaned and sanitized with caution, as they may contaminate other areas of the food processing facility. Cooking is a good way to protect meat from L. monocytogenes infection. The ideal processing procedure would extend the shelf life and safety of the meat product, without compromising its organoleptic or nutritional value, be simple and inexpensive to implement, and avoid causing consumers health risk [51].
Listeria contamination can be greatly reduced in many processed food products by using the Hazard Analysis of Critical Control Points (HACCP) system. To reduce listeriosis, different nations have adopted standards/legislations for the pasteurization of ice cream desserts [41].
Staphylococcus aureus. Characteristics. S. aureus is the second most frequent food-borne pathogen in the world after salmonellosis. It is a commensal microorganism that lives on the skin, nose, and mucous membranes of healthy people and animals. However, it is a well-known opportunistic food-borne pathogen that can cause a variety of infectious illnesses of varying severity in humans and animals. This microorganism can survive temperatures ranging from 7 to 48°C, with an optimum of 30 to 37°C, pH 4.2 to 9.3, with an optimum of 7.0 to 7.5, and sodium chloride concentrations up to 15% [53].
These features allow the bacterium to live in a wide range of foods, particularly those that require processing alteration, such as fermented products like cheese [54]. S. aureus is a desiccation-tolerant organism that may survive in potentially dry and stressful conditions like the human nose, as well as on the skin and inanimate surfaces like clothing [55].
Source and mode of transmission. Healthy animals carry germs in their nasal passages, throats, and skin, but S. aureus is commonly seen in raw milk from mastitic cows. It can be found in a wide range of hosts, including humans and food-producing animals like pigs, cows, goats, chickens, and ducks. Food contamination with S. aureus can arise directly from infected foodproducing animals or as a result of inadequate hygiene during the manufacturing process, as well as during retail and storage [53].
S. aureus is spread by contaminated animal-source foods [54]. Several food materials are regularly linked to staphylococcal food poisoning, including milk and dairy products, pork, beef, mutton, chicken, and eggs [53]. Raw meat provides a favorable medium for S. aureus survival and dissemination in the population, especially the drug-resistant S. aureus [56].
Methicillin-resistant S. aureus (MRSA) has been discovered at significant levels on US and European farms and in widely sold meats in various investigations, raising concerns among meat workers and consumers. Pig, poultry, and cattle are among those meat-producing animals which are usually implicated with the pathogen. According to a recent study conducted in the United States, 45% of raw pork products and 63% of beef products tested positive for S. aureus. A high prevalence of S. aureus was found on retail pork products in Iowa, Minnesota, and New Jersey. These investigations provide clue on the role of commercially-distributed meat as a possible vector for S. aureus transmission from farm to the general population [57].
In dairy herds, S. aureus is a common cause of bovine mastitis. The prevalence of Methicillin-susceptible S. aureus (MSSA) and MRSA was reported to be 84 and 4%, respectively, in a study conducted in Minnesota to determine the herd prevalence of S. aureus in bulk tank milk. Udders with clinical or subclinical staphylococcal mastitis can contribute to S. aureus contamination of milk by excreting the organisms directly into the milk. In a 1999 S. aureus incident in Brazil, for example, cattle mastitis was the primary source of infection, affecting 328 people who consumed unpasteurized milk. Similarly, 293 S. aureus isolates were found in 127 bulk tank milk samples from Swiss goats and sheep. Those findings revealed that the consumption of raw or improperly pasteurized milk poses a significant risk to public health [58].
Detection. The ability to produce coagulase is usually the most extensively utilized and widely accepted criterion for identifying pathogenic Staphylococci. Mannitol salt agar is a typical medium for the isolation of pathogenic Staphylococci. The current standard approach for detecting coagulase-positive Staphylococci in food is based on selective enrichment and subsequent isolation of colonies with distinctive morphology, followed by identification using microbiological and biochemical confirmations [54]. Immunological and molecular biology techniques are also useful tools for investigating S. aureus contaminations. For the quick detection and identification of MRSA strains, clinical laboratories are increasingly using polymerase chain reaction [53].
Prevention and control. Staphylococci are widespread and difficult to eradicate from the environment. To prevent staphylococcal infections and poisoning, methods to stop various mechanisms of transmission are required. Personal hygiene habits among healthcare professionals and food handlers should be improved. Equally effective are the decontamination of equipment, surfaces, and clothing, a judicious use of antibiotics, as well as proper cooking and storage of foods [54].
Staphylococcal infections can be prevented by avoiding contamination and cross-contamination, as well as maintaining critical points. Public education on safe meat handling and other public health interventions could be crucial in preventing the outbreak. Microbiological standards created by the World Health Organization and the US Food and Drug Administration, such as the Hazard Analysis and Critical Control Points (HACCP), Good Manufacturing Practices (GMPs), and good hygiene measures, should be closely followed to avoid S. aureus infection. Disinfectants should be used to clean areas where MRSA patients are cared for [57].
S. aureus can survive and produce toxins at temperatures ranging from 6 to 46°C. As a result, the ideal cooking and refrigeration temperatures are above 60°C and below 5°C, respectively. Other preventive measures include serving food quickly when kept at room temperature, controlling raw food products, disinfecting food processing and preparation equipment, wearing gloves, masks, and hairnets while handling and processing food, as well as frequent hand washing [59].
Escherichia. Characteristics. Escherichia is a Gramnegative rod-shaped bacterium that belongs to the Enterobacteriaceae family [60]. The majority of Escherichia coli are found in the gastrointestinal tracts of animals and humans, whereas some are harmful to humans [61]. E. coli is among the most common zoonotic species that poses a public health risk [62]. Several life-threatening food-borne epidemics have been linked to Shiga toxinproducing E. coli [63].
E. coli O157:H7 is one of the most well-known serotypes that can infect humans through the ingestion of infected animal foods [60]. It is a well-known Shiga toxin-producing bacterium that is a prominent food-borne and zoonotic pathogen [64].
Source and mode of transmission. Many healthy animals, including humans, have E. coli in their gut microbiota. Some strains, however, can cause sickness. Cattle, sheep, and goats are the main reservoirs of E. coli O157:H7. In outbreaks, milk and dairy products, as well as improperly cooked meat and other animal food products, have been identified as key sources of infection [65]. The consumption of contaminated animal food and water, fecal contamination of food products, and direct contact with sick animals are the most common routes of E. coli O157:H7 transmission to humans [66].
The global prevalence of E. coli O157:H7 in beef was found to range from 0.1 to 54.2% in ground beef, 0.1 to 4.4% in sausage, 1.1 to 36.0% in nonspecific retail cuts, and 0.01 to 43.4% in whole carcasses, according to a recent study. The first E. coli O157:H7 outbreak was reported in 1982, and it was connected to ground beef, which is still the most common source of food-borne outbreaks today. In the United States, beef was the cause of infection in 78 E. coli O157:H7-related outbreaks [67].
Raw milk is a potentially dangerous commodity whose microbiological safety cannot be ensured without pasteurization or a similar treatment. Cheeses prepared from raw milk are extremely prone to bacterial infection. If hygiene and process controls are weak, pathogens may enter cheese during production. Pathogens, such as E. coli O157:H7, can be found in raw milk, and mostly they come from milk animals resulting in human disease [68].
Detection. During outbreak investigations, sensitive methods, surveillance, and quality control are recommended to detect E. coli O157:H7 [66]. This serotype has been detected in food, environmental, and clinical samples using a variety of methods including culturing in specific media, as well as serological and molecular testing [69].
Biochemical assays and culture isolation of E. coli O157:H7 remain the gold standard for identification [70].
Sorbitol-MacConkey (SMAC) agar combined with cefixime and potassium tellurite is one of the most sensitive and discriminating mediums for separating E. coli O157:H7 from other E. coli serotypes [69]. Colorless colonies will appear if E. coli O157:H7 is present, but pink colonies will appear if other Enterobacteriaceae are present. Immunoassays and polymerase chain reaction (PCR) technology have made it possible to identify E. coli in food and water more quickly. Other techniques include enzyme-linked immunosorbent assays (ELISAs) [71].
Prevention and control. E. coli-related food poisoning can be avoided by using the same strategy as is used against other bacteria. Implementing intervention measures across the food continuum, from farm to table, will be necessary for an effective control program to significantly reduce E. coli O157:H7 infections. Consumers can also help implement intervention measures in the food handling and preparation process [72].
Pre-harvest E. coli O157:H7 control is a method that promotes human health, food, and water safety. The pathogen can also be reduced in animals by implementing proper sanitation procedures throughout food preparation, processing, and transportation management. Preventive measures include food hygiene such as correct meat cooking or intake of pasteurized milk, as well as personnel hygiene. With different degrees of success, post-harvest intervention strategies are used, such as skin and carcass cleaning and the application of antimicrobials [70].
The HACCP approach is used to ensure food safety in the processing industry. This method does not target E. coli specifically, but it addresses biological, chemical, and physical risks in general. Food handler training, food premise inspections, and community-based education programs were found to be effective in reducing public exposure to food-borne pathogens in a systematic review of retail and consumer food safety programs. Intervention approaches in cattle, such as probiotics, vaccination, antimicrobials, and bacteriophages, provide another option for increasing herd resistance to infection and subsequent control of E. coli O157:H7. Vaccination is a potential method of reducing E. coli O157:H7 colonization [70].
Brucella. Characteristics. Brucellosis is one of the important zoonotic diseases of livestock worldwide. The most important Brucella of veterinary and zoonotic importance that cause abortion and infertility in female and male livestock are Brucella abortus, Brucella melitensis, and Brucella suis [73]. The ability of the pathogen to survive and replicate within different host cells explains its pathogenicity. Its persistence in macrophages and other cell types leads to chronic infections [74].
Source and mode of transmission. In animals, the transmission of Brucella can occur either by direct or indirect interaction with diseased cattle or their discharges. The most common route is ingesting feed and drinking water contaminated by the bacteria that are present in massive amounts in birth products and uterine discharge. Moreover, cattle typically lick their fetuses and newborn calves, which can result in bacterial transmission to humans [75].
Humans typically acquire Brucella infection by ingesting raw infected meat, unpasteurized milk, or dairy products. They can also get infected through abrasions that come into contact with fluids or tissues of aborted fetuses of diseased cattle, as well as other work-related practices. Abattoir, farm, and laboratory workers, as well as veterinarians, are known risk groups for Brucella infection [76].
Detection. The test samples from which DNA can be extracted most commonly for brucellosis diagnosis include aborted fetuses and food products, such as milk and other foods. The most reliable samples in animals for Brucella isolation are spleen as well as lymph nodes (iliac, mammary, and pre-femoral) during the postmortem inspection [77].
O’Grady et al. compared cultural and serological techniques to isolate B. abortus from paired supra-mammary, retropharyngeal, and internal iliac lymph nodes of slaughtered animals [78]. Although the micro serum agglutination test and the fluorescence polarization assay were found to be comparatively more sensitive, bacterial culture methods should always be employed for Brucella confirmation [79].
Prevention and control. Brucellosis in animals may be controlled by a strict enforcement of measures including testing and slaughtering, vaccination, sanitation, and movement control. The knowledge of brucellosis and its presence in different livestock species is essential for the effective implementation of control measures in food safety. Quarantine and immunization of animals with vaccines are also among important measures of prevention and control [77].
The lack of awareness among farmers and the absence of an effective control strategy have made the situation favorable for Brucella. Therefore, animal owners should be made aware of the health impact of brucellosis and the importance of vaccinating their livestock. Public health education should emphasize food and occupational hygiene. Avoiding or discouraging the consumption of raw milk and dairy products, as well as strict protection and safety measures among health workers, will help prevent brucellosis in the human population [80].
Clostridium botulinum. Characteristics. C. botulinum is a bacterium that produces a dangerous toxin which is reported to be one of the most known lethal substances [81]. C. botulinum can colonize the intestinal tract of animals and produce botulism neurotoxin. During unfavorable conditions, C. botulinum forms a spore that survives standard cooking and food-processing measures. However, the spore is sensitive to an aerobic environment, acidic pH, and high salt solutions [82]. Spore germination and toxin production are achieved when foods are exposed to anaerobic conditions, pH > 4.6, low salt and sugar concentrations, and temperatures from 4–12°C [83].
Source and mode of transmission. Botulism is known to affect mammals, birds, and fish, although some species seem to be more susceptible than others. This disease is reported regularly in horses, domesticated ruminants, poultry, wild birds, and aquatic animals. Botulism is not transmitted between animals or people by casual contact. However, it can be acquired by ingesting preformed toxins. Humans contract the toxin by ingesting contaminated livestock products [84].
C. botulinum strains are responsible for numerous botulism outbreaks in humans. Uncooked or minimally heated, chilled livestock products are often involved in the transmission of botulism to humans. C. botulinum is a safety risk in home-made or industrial foods that are uncooked or processed with mild heat treatments, as well as stored for long periods even at low temperatures [85]. Meat can be contaminated with fecal C. botulinum spores during processing at the slaughter house. Pork meat preparations carry an important risk of botulism in several countries such as France and Poland. In France, type B botulism caused by home-made preparations of pork meat is the most prevalent food-borne botulism [86].
Raw milk contamination by C. botulinum results essentially from the cattle environment. Indeed, in farms with a botulism outbreak, the diseased as well as non-symptomatic animals excrete large numbers of C. botulinum spores in their feces that are subsequently spread in their local environment, including soil and pasture. Diseased cows are unlikely to excrete botulinum neurotoxin in milk. The toxin is very rarely detected in the serum of cattle with botulism. Thus, the passage of a significant amount of botulinum neurotoxin into milk is very low, with very few outbreaks of human botulism via milk products reported. A report by Dąbrowski and Mędrala shows the presence of botulinum neurotoxin B in the milk of a cow suffering from mastitis [87].
Detection. Food-borne botulism is initially suspected based on the clinical case of the patient. It can be confirmed through a laboratory identification of botulinum neurotoxin and/or those clostridia that produce the toxin in clinical specimens or in suspected food sources consumed by the patient [87].
Several methods are used to detect the toxin. The intra-peritoneal injection of the bacteria to mice remains the most reliable, sensitive, and definitive of all the methods. The presence of C. botulinum is detected by injecting the food extract into mice, which are then observed for characteristic symptoms of botulism and ultimate death over a 48-h period. The mouse bioassay is the only standard validated method for the detection of botulinum toxin in foods [88].
Prevention and control. The toxins are typically sensitive and their spores can be destroyed by heating to 85°C for 5 min or by wet sterilization at 120°C for 5 min. Veterinarians who encounter or suspect botulism should follow their national and/or local guidelines for disease reporting [89].
Feed for animals may be heat-processed and acidified to reduce the risk of botulism. Carcasses should not be allowed to contaminate feed for herbivores, and silage should be monitored for proper acidification. Reusing broiler litter on ruminant farms as feed or bedding increases the risk of botulism. When broiler litter is spread on fields, it should be plowed in immediately. Ruminants with dietary deficiencies should be given feed supplements to reduce the incidence of pica. Vaccines may be available for horses, cattle, sheep, goats, mink, and/or birds in some countries [89].
In humans, the risk of botulism from foods can also be reduced by acidification, moisture reduction, and treatment with salt or other compounds known to inhibit clostridial germination before consumption. Humans should avoid foods from animals with botulism, including meat and milk. Person-to-person transmission of botulinum has never been described, but precautions should be taken to avoid exposure to toxins in body fluids and feces [57].
Clostridium perfringens. Characteristics. C. perfringens belongs to the Bacillicidal family and is an important cause of food-borne disease. It produces protein toxins and forms spores resistant to various environmental stresses such as radiation, desiccation, and heat. Vegetative cells grow at temperatures ranging from 6 to 50°C but prefer an optimum temperature between 43 and 47°C, a salt concentration less than 5–8% depending on the strain, and a pH of 5.0–9.0, although 6.0–7.2 is preferred. C. perfringens is part of the normal intestinal flora of animals and humans [90].
Source and mode of transmission. C. perfringens thrives in high-protein foods of animal origin, such as meat and meat products, meat dishes, and milk. These protein-containing foods, when kept at improper storage temperatures between 12 and 60°C, provide the greatest risk of infection and disease from C. perfringens. This is because its spores present after cooking can germinate and potentially grow to high numbers. The risk zone is between 43 and 47°C [91].
Foods can be contaminated during food production, processing, preparation, transportation, and storage. The initial contamination of foods implicated in C. perfringens outbreaks might have occurred in the environment, since C. perfringens is ubiquitous in soil, in intestinal tracts of animals, and in a variety of ingredients. Raw meat and dairy products can become contaminated if the animal is infected. If consumed raw or after insufficient cooking, these foods may result in bacterial proliferation and toxin production [90].
Detection. Food samples are inoculated into Rapid Perfringens Media (RPM) overnight, then sandwiched into tryptose sulphite cycloserine (TSC) agar, and incubated. Colonies suspected to be C. perfringens are then sub-cultured into RPM and incubated again. Typical C. perfringens colonies from agar plates are selected and inoculated into a tube of freshly deaerated and cooled fluid thioglycollate broth. They are incubated in a standard incubator at 35°C for 18–24 h. Then, the cultures are examined by Gram stain and checked for purity. C. perfringens is a short, thick, yellowish gray Gram-positive bacillus. DNA is extracted and PCR can also be performed to determine its genotype [92].
Prevention and control. Thermal treatment is one of the most common ways of sterilizing products, as excessive heat destroys the majority of bacterial cells. It has been reported that C. perfringens spores are highly heat-resistant, although the resistance patterns vary considerably with the strain and growth conditions, such as the medium and incubation temperature. However, a significant inactivation of C. perfringens spores can be achieved by exposing them to high temperatures for a longer period of time. Additionally, cooked food must be chilled rapidly to 41°F (5°C) or less, or kept at hot holding temperatures of 140°F (60°C) or higher to prevent any activation and growth of C. perfringens spores [93].
Different types of chemical agents have been proven to be effective in controlling C. perfringens such as acetic acid, organic acids, nitrates, and phosphates. In animals, vaccination can be used to control C. perfringens B and C [94].
Mycobacterium bovis. Characteristics. The Mycobacterium genus includes non-spore forming, obligate aerobic, facultative intracellular species. The bacteria’s cell walls are rich in lipid, which greatly contributes to their resistance to many disinfectants, common laboratory stains, and antibiotics [95]. M. bovis is the main etiological agent of bovine tuberculosis. While the bacteria primarily affect the cattle and other domestic and wild animals, they can also affect human beings [96].
Source and mode of transmission. M. bovis is one of the bacteria recognized as a major public health concern. M. bovis is the cause of zoonotic tuberculosis in humans, which can be transmitted to people from infected vertebrate animals. It is the most common cause of tuberculosis in humans and cattle [97]. Inhalation and ingestion are two common modes of transmission. Inhalation transmission is mainly by droplet infection. Animals and humans can inhale dust contaminated by sputum, feces, or urine of infected animals. Thus, close housing and overcrowding, along with improper management, increase the risk of the disease in animals. Infected cattle are a possible source of infection as they shed a significant amount of M. bovis through droplets into the environmental. They may act as a source of intra-herd transmission either directly (animal-to-animal), particularly by aerosol, or indirectly, via infective material (e.g., manure, urine, bedding, contaminated feed, and water) [96].
M. bovis is transmitted from animal to human through ingestion of unpasteurized dairy products and undercooked meat of infected cattle [96]. In an estimate, about 10% of human tuberculosis cases are caused by M. bovis, while the majority are caused by M. tuberculosis. In the countries where milk is pasteurized and effective bovine tuberculosis control is implemented, tuberculosis occurrence in humans due to M. bovis is very rare. However, in the areas where the disease in bovine is poorly controlled, the disease reports are more frequent [98].
Detection. Different tests can be undertaken to detect M. bovis in food samples. The identification of M. bovis in raw milk has been described in Tanzania. Microbiological culture remains the gold standard test for detecting Mycobacterium species in clinical samples due to its sensitivity and specificity. When tuberculosis lesions are found in a carcass during meat inspection at routine slaughter, a sample of the affected tissue is also sent for mycobacterial culture testing [99].
Enrichment selective media are required to isolate Mycobacterium species, especially those containing egg, which is commonly used in veterinary microbiology. One of them is the Lowenstein-Jensen medium made with egg and glycerol favors. After culture, other tests are carried out, such as species identification, antimicrobial susceptibility profile, genotyping, as well as monitoring the human patient’s response to treatment [100].
Prevention and control. Tuberculosis needs to be prevented and controlled because it causes a loss of productivity in infected animals and poses a risk of infection to humans. However, it is still a big problem in most developing countries because of financial constraints, scarcity of trained professionals, and lack of political will. Moreover, national governments and donor agencies seem to underestimate the importance of zoonotic tuberculosis in both animal and public health sectors. Therefore, control measures are not applied or applied inadequately [101].
Cattle with tuberculosis must be slaughtered, rather than treated. This is due to the fact that M. bovis is resistant to pyrazinamide, which is widely used in the treatment of infections caused by Mycobacterium tuberculosis in humans. Meat inspection systems should be strengthened and designed to prevent the consumption of contaminated products by people. All animals entering the food chain should be subjected to antemortem and post-mortem inspection. The tuberculin test is valuable in the control of zoonotic tuberculosis because early recognition of preclinical infection in the animals intended for food production eliminates a future source of infection for humans [102].
Standard public health measures used to manage patients with contagious M. tuberculosis should be applied to contagious patients with M. bovis to stop person-to-person transmission. Hygienic measures should be instituted to prevent the spread of infection. Food containers should be cleaned and thoroughly disinfected, while the consumption of unpasteurized milk, as well as undercooked or raw meat, should be avoided [96].
ВЫВОДЫ
Food-borne zoonotic bacterial infections are the primary causes of human illness worldwide, with a high burden in developing countries. Campylobacter, Salmonella, Listeria, Staphylococcus, Escherichia, Brucella, Clostridium, and Mycobacterium are the most implicated bacterial pathogens to be found in animal products either from intrinsic infection or environmental contamination with the pathogens or their toxins. Moreover, these bacteria may also infiltrate into the food chain at any stage and affect public health. More importantly, due to the rise of multidrug-resistant strains, most of the bacterial diseases cannot be cured with previous conventional medicaments, exacerbating the problem.
International scientists have regularly isolated the bacteria from food of animal origin, particularly meat, dairy products, and eggs. People may be more susceptible to food-borne zoonotic bacterial infections if they consume raw livestock products, meat supplied from poorly standardized slaughter houses, or use unsanitary food preparation and handling procedures. This calls for a vigorous implementation of effective prevention and control strategies in all food supply chains to ensure food safety. To this effect, the following recommendations have been put forward:
– coordinated surveillance and monitoring systems for food-borne zoonotic bacterial pathogens should be applied to alleviate their impact on public health;
– the epidemiological information system should be established to cover potential risk factors and incidence of human infections associated with food-borne zoonotic bacterial pathogens; and
– awareness should be raised in the community have the occurrence to prevent and control of food-borne zoonotic bacterial pathogens of livestock origin in humans.
Вклад авторов
Fuad Zenu collected the data and wrote the paper. Tesfaye Bekele conceived conceptual frame work and designed the analysis and wrote the paper.
КОНФЛИКТ ИНТЕРЕСОВ
The authors declare no conflict of interest.
СПИСОК ЛИТЕРАТУРЫ
- Phelps LN, Kaplan JO. Land use for animal production in global change studies: Defining and characterizing a framework. Global Change Biology. 2017;23(11):4457–4471. https://doi.org/10.1111/gcb.13732
- Balehegn M, Kebreab E, Tolera A, Hunt S, Erickson P, Crane TA, et al. Livestock sustainability research in Africa with a focus on the environment. Animal Frontiers. 2021;11(4):47–56. https://doi.org/10.1093/af/vfab034
- Rojas-Downing MM, Nejadhashemi AP, Harrigan T, Woznicki SA. Climate change and livestock: Impacts, adaptation, and mitigation. Climate Risk Management. 2017;16:145–163. https://doi.org/10.1016/j.crm.2017.02.001
- Moyo S, Swanepoel F, Stroebel A. The role of livestock in developing communities: Enhancing multifunctionality. UJ Press; 2010. 236 p.
- Eshetie T, Hussien K, Teshome T, Mekonnen A. Meat production, consumption and marketing tradeoffs and potentials in Ethiopia and its effect on GDP growth: A review. Journal of Nutritional Health and Food Engineering. 2018;8(3):228–233. https://doi.org/10.15406/jnhfe.2018.08.00274
- Hemalata VB, Virupakshaiah DBM. Isolation and identification of food borne pathogens from spoiled food samples. International Journal of Current Microbiology and Applied Sciences. 2016;5(6):1017–1025. https://doi.org/10.20546/ijcmas.2016.506.108
- Recht J, Schuenemann VJ, Sánchez-Villagra MR. Host diversity and origin of zoonoses: The ancient and the new. Animals. 2020;10(9). https://doi.org/10.3390/ani10091672
- Grujić S, Grujčić M. Factors affecting consumer preference for healthy diet and functional foods. Foods and Raw Materials. 2023;11(2):259–271. https://doi.org/10.21603/2308-4057-2023-2-576
- Abebe M, Hailelule A, Abrha B, Nigus A, Birhanu M, Adane H, et al. Antibiogram of Escherichia coli strains isolated from food of bovine origin in selected Woredas of Tigray, Ethiopia. Journal of Bacteriology Research. 2014;6(3):17–22.
- Haileselassie M, Taddele H, Adhana K, Kalayou S. Food safety knowledge and practices of abattoir and butchery shops and the microbial profile of meat in Mekelle City, Ethiopia. Asian Pacific Journal of Tropical Biomedicine. 2013;3(5):407–412. https://doi.org/10.1016/S2221-1691(13)60085-4
- Dhama K, Rajagunalan S, Chakraborty S, Verma AK, Kumar A, Tiwari R, et al. Food-borne pathogens of animal origin-diagnosis, prevention, control and their zoonotic significance: A review. Pakistan Journal of Biological Sciences. 2013;16(20):1076–1085. https://doi.org/10.3923/pjbs.2013.1076.1085
- Heredia N, García S. Animals as sources of food-borne pathogens: A review. Animal Nutrition. 2018;4(3):250–255. https://doi.org/10.1016/j.aninu.2018.04.006
- Zhao X, Lin C-W, Wang J, Oh DH. Advances in rapid detection methods for foodborne pathogens. Journal of Microbiology and Biotechnology. 2014;24(3):297–312. https://doi.org/10.4014/jmb.1310.10013
- Zelalem A, Sisay M, Vipham JL, Abegaz K, Kebede A, Terefe Y. The prevalence and antimicrobial resistance profiles of bacterial isolates from meat and meat products in Ethiopia: A systematic review and meta-analysis. International Journal of Food Contamination. 2019;6. https://doi.org/10.1186/s40550-019-0071-z
- Addis M, Sisay D. A review on major food borne bacterial illnesses. Journal of Tropical Diseases. 2015;3.
- Kebede T, Afera B, Taddele H, Bsrat A. Assessment of bacteriological quality of sold meat in the butcher shops of Adigrat, Tigray, Ethiopia. Applied Journal of Hygiene. 2014;3(3):38–44.
- Dadi L, Asrat D. Prevalence and antimicrobial susceptibility profiles of thermotolerant Campylobacter strains in retail raw meat products in Ethiopia. Ethiopian Journal of Health Development. 2008;22(2):195–200. https://doi.org/10.4314/ejhd.v22i2.10072
- Wieczorek K, Wołkowicz T, Osek J. Antimicrobial resistance and virulence-associated traits of Campylobacter jejuni isolated from poultry food chain and humans with diarrhea. Frontiers in Microbiology. 2018;9. https://doi.org/10.3389/fmicb.2018.01508
- Jacobs-Reitsma WF, Newell DG, Wagenaar JA. Campylobacter jejuni and Campylobacter coli. In: Manual of diagnostic tests and vaccines for terrestrial animals. Paris: Office International des Epizooties; 2008. pp. 1185–1191.
- Young KT, Davis LM, DiRita VJ. Campylobacter jejuni: Molecular biology and pathogenesis. Nature Reviews Microbiology. 2007;5:665–679. https://doi.org/10.1038/nrmicro1718
- Andrzejewska M, Szczepańska B, Śpica D, Klawe JJ. Prevalence, virulence, and antimicrobial resistance of Campylobacter spp. in raw milk, beef, and pork meat in Northern Poland. Foods. 2019;8(9). https://doi.org/10.3390/foods8090420
- Fernandes AM, Balasegaram S, Willis C, Wimalarathna HML, Maiden MC, McCarthy ND. Partial failure of milk pasteurization as a risk for the transmission of Campylobacter from cattle to humans. Clinical Infectious Diseases. 2015;61(6):903–909. https://doi.org/10.1093/cid/civ431
- Taylor EV, Herman KM, Ailes EC, Fitzgerald C, Yoder JS, Mahon BE, et al. Common source outbreaks of Campylobacter infection in the USA, 1997–2008. Epidemiology and Infection. 2013;141(5):987–996. https://doi.org/10.1017/S0950268812001744
- Jay-Russell MT, Mandrell RE, Yuan J, Bates A, Manalac R, Mohle-Boetani J, et al. Using major outer membrane protein typing as an epidemiological tool to investigate outbreaks caused by milk-borne Campylobacter jejuni isolates in California. Journal of Clinical Microbiology. 2013;51(1):195–201. https://doi.org/10.1128/JCM.01845-12
- Progress on sanitation and drinking water: 2015 update and MDG assessment. UNICEF and World Health Organization; 2015. 90 p.
- Humphrey S, Chaloner G, Kemmett K, Davidson N, Williams N, Kipar A, et al. Campylobacter jejuni is not merely a commensal in commercial broiler chickens and affects bird welfare. mBio. 2014;5(4). https://doi.org/10.1128/mBio.01364-14
- Lake RJ, Horn BJ, Dunn AH, Parris R, Green FT, McNickle DC. Cost-effectiveness of interventions to control Campylobacter in the New Zealand poultry meat food supply. Journal of Food Protection. 2013;76(7):1161–1167. https://doi.org/10.4315/0362-028X.JFP-12-481
- Chen J, Sun X-T, Zeng Z, Yu Y-Y. Campylobacter enteritis in adult patients with acute diarrhea from 2005 to 2009 in Beijing, China. Chinese Medical Journal. 2011;124(10):1508–1512. https://doi.org/10.3760/cma.j.issn.0366-6999.2011.10.013
- Mogasale V, Maskery B, Ochiai RL, Lee JS, Mogasale VV, Ramani E, et al. Burden of typhoid fever in low-income and middle-income countries: A systematic, literature-based update with risk-factor adjustment. The Lancet Global Health. 2014;2(10):e570–e580. https://doi.org/10.1016/S2214-109X(14)70301-8
- Evdokimova SA, Karetkin BA, Zhurikov MO, Guseva EV, Khabibulina NV, Shakir IV, et al. Antagonistic activity of synbiotics: Response surface modeling of various factors. Foods and Raw Materials. 2022;10(2):365–376. https://doi.org/10.21603/2308-4057-2022-2-543
- Gal-Mor O, Boyle EC, Grassl GA. Same species, different diseases: how and why typhoidal and non-typhoidal Salmonella enterica serovars differ. Frontiers in Microbiology. 2014;5. https://doi.org/10.3389/fmicb.2014.00391
- Johnson TJ, Wannemuehler YM, Johnson SJ, Logue CM, White DG, Doetkott C, et al. Plasmid replicon typing of commensal and pathogenic Escherichia coli isolates. Applied and Environmental Microbiology. 2007;73(6):1976–1983. https://doi.org/10.1128/AEM.02171-06
- Kemal J. A review on the public health importance of bovine salmonellosis. Journal of Veterinary Science and Technology. 2014;5. https://doi.org/10.4172/2157-7579.1000175
- Radostits OM, Gay CC, Hinchcliff KW, Constable PD. Veterinary medicine: A textbook of the diseases of cattle, horses, sheep, pigs and goats. Saunders Ltd.; 2007. 2180 p.
- Kemal J, Sibhat B, Menkir S, Terefe Y, Muktar Y. Antimicrobial resistance patterns of Salmonella in Ethiopia: A review. African Journal of Microbiology Research. 2015;9(46):2249–2256. https://doi.org/10.5897/AJMR2015.7763
- Girma G. Prevalence, antibiogram and growth potential of Salmonella and Shigella in Ethiopia: Implications for public health: A review. Research Journal of Microbiology. 2015;10(7):288–307. https://doi.org/10.3923/jm.2015.288.307
- Jones BD. Salmonella invasion gene regulation: A story of environmental awareness. Journal of Microbiology. 2005;43:110–117.
- Technical specifications on the harmonised monitoring and reporting of antimicrobial resistance in Salmonella, Campylobacter and indicator Escherichia coli and Enterococcus spp. bacteria transmitted through food. EFSA Journal. 2012;10(6). https://doi.org/10.2903/j.efsa.2012.2742
- Lee K-M, Runyon M, Herrman TJ, Phillips R, Hsieh J. Review of Salmonella detection and identification methods: Aspects of rapid emergency response and food safety. Food control. 2015;47:264–276. https://doi.org/10.1016/j.foodcont.2014.07.011
- Wang J, Li Y, Chen J, Hua D, Li Y, Deng H, et al. Rapid detection of food-borne Salmonella contamination using IMBs-qPCR method based on pagC gene. Brazilian Journal of Microbiology. 2018;49(2):320–328. https://doi.org/10.1016/j.bjm.2017.09.001
- Pal M, Awel H. Public health significance of Listeria monocytogenes in milk and milk products: An overview. Journal of Veterinary Public Health. 2014;12(1):1–5.
- Lee SHI, Cappato LP, Guimarães JT, Balthazar CF, Rocha RS, Franco LT, et al. Listeria monocytogenes in milk: Occurrence and recent advances in methods for inactivation. Beverages. 2019;5(1). https://doi.org/10.3390/beverages5010014
- Shamloo E, Abdimoghadam Z, Nazari K, Hosseini SM, Hosseini H, Alebouyeh M. Long term survival of Listeria monocytogenes in stress conditions: High pH and salt concentrations. Journal of Research in Medical and Dental Science. 2018;6(6):96–100.
- Colagiorgi A, Bruini I, Di Ciccio PA, Zanardi E, Ghidini S, Ianieri A. Listeria monocytogenes biofilms in the wonderland of food industry. Pathogens. 2017;6(3). https://doi.org/10.3390/pathogens6030041
- Al-mashhadany DA, Ba-Salamah HA, Shater A-R, Al Sanabani AS, Abd Al Galil FM. Prevalence of Listeria monocytogenes in red meat in Dhamar Governorate/Yemen. Prevalence. 2016;2(12):73–78.
- Şanlıbaba P, Tezel BU. Prevalence and characterization of Listeria species from raw milk and dairy products from Çanakkale province. Turkish Journal of Agriculture – Food Science and Technology. 2018;6(1):61–64. https://doi.org/10.24925/turjaf.v6i1.61-64.1641
- Girma Y, Abebe B. Isolation, identification and antimicrobial susceptibility of Listeria species from raw bovine milk in Debre-Birhan Town, Ethiopia. Journal of Zoonotic Diseases and Public Health. 2018;2(1).
- Analysis of the baseline survey on the prevalence of Listeria monocytogenes in certain ready‐to‐eat foods in the EU, 2010–2011 Part A: Listeria monocytogenes prevalence estimates. EFSA Journal. 2013;11(6). https://doi.org/10.2903/j.efsa.2013.3241
- Bolocan AS, Oniciuc EA, Alvarez-Ordonez A, Wagner M, Rychli K, Jordan K, et al. Putative cross-contamination routes of Listeria monocytogenes in a meat processing facility in Romania. Journal of Food Protection. 2015;78(9):1664–1674. https://doi.org/10.4315/0362-028X.JFP-14-539
- Law JW-F, Ab Mutalib N-S, Chan K-G, Lee L-H. An insight into the isolation, enumeration, and molecular detection of Listeria monocytogenes in food. Frontiers in Microbiology. 2015;6. https://doi.org/10.3389/fmicb.2015.01227
- Rajkovic A, Smigic N, Devlieghere F. Contemporary strategies in combating microbial contamination in food chain. International Journal of Food Microbiology. 2010;141:S29–S42. https://doi.org/10.1016/j.ijfoodmicro.2009.12.019
- Dhama K, Karthik K, Tiwari R, Shabbir MZ, Barbuddhe S, Malik SVS, et al. Listeriosis in animals, its public health significance (food-borne zoonosis) and advances in diagnosis and control: A comprehensive review. Veterinary Quarterly. 2015;35(4):211–235. https://doi.org/10.1080/01652176.2015.1063023
- Wang W, Baloch Z, Jiang T, Zhang C, Peng Z, Li F, et al. Enterotoxigenicity and antimicrobial resistance of Staphylococcus aureus isolated from retail food in China. Frontiers in Microbiology. 2017;8. https://doi.org/10.3389/fmicb.2017.02256
- Argaw S, Addis M. A review on staphylococcal food poisoning. Food Science and Quality Management. 2015;40:59–72.
- Chaibenjawong P, Foster SJ. Desiccation tolerance in Staphylococcus aureus. Archives of Microbiology. 2011;193:125–135. https://doi.org/10.1007/s00203-010-0653-x
- Abebe E, Gugsa G, Ahmed M. Review on major food-borne zoonotic bacterial pathogens. Journal of Tropical Medicine. 2020;2020. https://doi.org/10.1155/2020/4674235
- Smith TC, Male MJ, Harper AL, Kroeger JS, Tinkler GP, Moritz ED, et al. Methicillin-resistant Staphylococcus aureus (MRSA) strain ST398 is present in midwestern US swine and swine workers. PLoS ONE. 2009;4(1). https://doi.org/10.1371/journal.pone.0004258
- do Carmo LS, Dias RS, Linardi VR, de Sena MJ, dos Santos DA, de Faria ME, et al. Food poisoning due to enterotoxigenic strains of Staphylococcus present in Minas cheese and raw milk in Brazil. Food Microbiology. 2002;19(1):9–14. https://doi.org/10.1006/fmic.2001.0444
- James SJ, Evans J, James C. A review of the performance of domestic refrigerators. Journal of Food Engineering. 2008;87(1):2–10. https://doi.org/10.1016/j.jfoodeng.2007.03.032
- Assefa A, Bihon A. A systematic review and meta-analysis of prevalence of Escherichia coli in foods of animal origin in Ethiopia. Heliyon. 2018;4(8). https://doi.org/10.1016/j.heliyon.2018.e00716
- Bekele T, Zewde G, Tefera G, Feleke A, Zerom K. Escherichia coli O157: H7 in raw meat in Addis Ababa, Ethiopia: Prevalence at an abattoir and retailers and antimicrobial susceptibility. International Journal of Food Contamination. 2014;1. https://doi.org/10.1186/s40550-014-0004-9
- Geresu MA, Regassa S. Escherichia coli O157: H7 from food of animal origin in Arsi: Occurrence at catering establishments and antimicrobial susceptibility profile. The Scientific World Journal. 2021;2021. https://doi.org/10.1155/2021/6631860
- Ahmida MR. Molecular identification of certain virulence genes of some food poisoning bacteria contaminating raw milk. Damanhour Journal of Veterinary Sciences. 2020;4(2):20–24.
- Haile AF, Kebede D, Wubshet AK. Prevalence and antibiogram of Escherichia coli O157 isolated from bovine in Jimma, Ethiopia: Abattoirbased survey. Ethiopian Veterinary Journal. 2017;21(2):109–120. https://doi.org/10.4314/evj.v21i2.8
- Messele YE. Characterization of drug resistance patterns of E. coli isolated from milk collected from small scale dairy farms reared in Holeta and Burayu and meat from Addis Ababa abattoirs enterprise and Alema farm slaughter slab. Doctoral dissertation. Addis Ababa University; 2016. 68 p.
- Abreham S, Teklu A, Cox E, Sisay Tessema T. Escherichia coli O157:H7: Distribution, molecular characterization, antimicrobial resistance patterns and source of contamination of sheep and goat carcasses at an export abattoir, Mojdo, Ethiopia. BMC microbiology. 2019;19. https://orcid.org/0000-0002-4103-5029
- Lu Z, Breidt F. Escherichia coli O157:H7 bacteriophage Φ241 isolated from an industrial cucumber fermentation at high acidity and salinity. Frontiers in Microbiology. 2015;6. https://doi.org/10.3389/fmicb.2015.00067
- Fusco V, Chieffi D, Fanelli F, Logrieco AF, Cho G-S, Kabisch J, et al. Microbial quality and safety of milk and milk products in the 21st century. Comprehensive Reviews in Food Science and Food Safety. 2020;19(4):2013–2049. https://doi.org/10.1111/1541-4337.12568
- Farahmandfar M, Moori-Bakhtiari N, Gooraninezhad S, Zarei M. Comparison of two methods for detection of E. coli O157H7 in unpasteurized milk. Iranian Journal of Microbiology. 2016;8(5):282–287.
- Saeedi P, Yazdanparast M, Behzadi E, Salmanian AH, Mousavi SL, Nazarian S, et al. A review on strategies for decreasing E. coli O157:H7 risk in animals. Microbial Pathogenesis. 2017;103:186–195. https://doi.org/10.1016/j.micpath.2017.01.001
- Mesele F, Abunna F. Escherichia coli O157:H7 in foods of animal origin and its food safety implications: Review. Advances in Biological Research. 2019;13(4):134–145.
- Pennington H. Escherichia coli O157. The Lancet. 2010;376(9750):1428–1435. https://doi.org/10.1016/S0140-6736(10)60963-4
- Godfroid J, Scholz HC, Barbier T, Nicolas C, Wattiau P, Fretin D, et al. Brucellosis at the animal/ecosystem/human interface at the beginning of the 21st century. Preventive Veterinary Medicine. 2011;102(2):118–131. https://doi.org/10.1016/j.prevetmed.2011.04.007
- Ray K, Marteyn B, Sansonetti PJ, Tang CM. Life on the inside: The intracellular lifestyle of cytosolic bacteria. Nature Reviews Microbiology. 2009;7:333–340. https://doi.org/10.1038/nrmicro2112
- Tulu D, Deresa B, Begna F, Gojam A. Review of common causes of abortion in dairy cattle in Ethiopia. Journal of Veterinary Medicine and Animal Health. 2018;10(1):1–3. https://doi.org/10.5897/JVMAH2017.0639
- Fugier E, Pappas G, Gorvel J-P. Virulence factors in brucellosis: implications for aetiopathogenesis and treatment. Expert Reviews in Molecular Medicine. 2007;9(35):1–10. https://doi.org/10.1017/S1462399407000543
- Etter RP, Drew ML. Brucellosis in elk of eastern Idaho. Journal of Wildlife Diseases. 2006;42(2):271–278. https://doi.org/10.7589/0090-3558-42.2.271
- O'Grady J, Ruttledge M, Sedano-Balbas S, Smith TJ, Barry T, Maher M. Rapid detection of Listeria monocytogenes in food using culture enrichment combined with real-time PCR. Food Microbiology. 2009;26(1):4–7. https://doi.org/10.1016/j.fm.2008.08.009
- Ledwaba MB, Ndumnego OC, Matle I, Gelaw AK, van Heerden H. Investigating selective media for optimal isolation of Brucella spp. in South Africa. Onderstepoort Journal of Veterinary Research. 2020;87(1). https://doi.org/10.4102/ojvr.v87i1.1792
- Acharya KP, Kaphle K, Shrestha K, Garin Bastuji B, Smits HL. RETRACTED: Review of brucellosis in Nepal. International Journal of Veterinary Science and Medicine. 2016;4(2):54–62. https://doi.org/10.1016/j.ijvsm.2016.10.009
- Kamaloddini MH, Kheradmand HR. A foodborne botulism occurrence in Mashhad: Clostridium botulinum in local cheese. Journal of Emergency Practice and Trauma. 2021;7(1):66–68. https://doi.org/10.34172/jept.2020.01
- Sobel J, Tucker N, Sulka A, McLaughlin J, Maslanka S. Foodborne botulism in the United States, 1990–2000. Emerging Infectious Diseases. 2004;10(9)1606–1611. https://doi.org/10.3201/eid1009.030745
- Chaidoutis E, Keramydas D, Papalexis P, Migdanis A, Migdanis I, Lazaris AC, et al. Foodborne botulism: A brief review of cases transmitted by cheese products (Review). Biomedical Reports. 2022;16(5). https://doi.org/10.3892/br.2022.1524
- Abe Y, Negasawa T, Monma C, Oka A. Infantile botulism caused by Clostridium butyricum type E toxin. Pediatric Neurology. 2008;38(1):55–57. https://doi.org/10.1016/j.pediatrneurol.2007.08.013
- Rasetti-Escargueil C, Lemichez E, Popoff MR. Public health risk associated with botulism as foodborne zoonoses. Toxins. 2020;12(1). https://doi.org/10.3390/toxins12010017
- Mazuet C, da Silva NJ, Legeay C, Sautereau J, Popoff RM. Human botulism in France, 2013–2016. Bulletin Epidémiologique Hebdomadaire. 2018;3:46–54. (In French).
- Dąbrowski W, Mędrala D. Bacterial toxins. In: Dabrowski WM, Sikorski ZE, editors. Toxins in food. Boca Raton: CRC Press; 2004. https://doi.org/10.1201/9780203502358
- van Baar BLM, Hulst AG, de Jong AL, Wils ERJ. Characterisation of botulinum toxins type C, D, E, and F by matrix-assisted laser desorption ionisation and electrospray mass spectrometry. Journal of Chromatography A. 2004;1035(1):97–114.
- Madsen JM. Bio warfare and terrorism: toxins and other mid-spectrum agents. Army medical research inst of chemical defense aberdeen proving ground; 2005. 8 p.
- Grass JE, Gould LH, Mahon BE. Epidemiology of foodborne disease outbreaks caused by Clostridium perfringens, United States, 1998–2010. Foodborne Pathogens and Disease. 2013;10(2):131–136. https://doi.org/10.1089/fpd.2012.1316
- Gui L, Subramony C, Fratkin J, Hughson MD. Fatal enteritis necroticans (pigbel) in a diabetic adult. Modern Pathology. 2002;15(1):66–70. https://doi.org/10.1038/modpathol.3880491
- Johansson A, Engström BE, Frey J, Johansson K-E, Båverud V. Survival of Clostridium perfringens during simulated transport and stability of some plasmid-borne toxin genes under aerobic conditions. Acta Veterinaria Scandinavica. 2005;46. https://doi.org/10.1186/1751-0147-46-241
- Wang G, Paredes‐Sabja D, Sarker MR, Green C, Setlow P, Li Y-Q. Effects of wet heat treatment on the germination of individual spores of Clostridium perfringens. Journal of Applied Microbiology. 2012;113(4):824–836. https://doi.org/10.1111/j.1365-2672.2012.05387.x
- Jay JM, Loessner MJ, Golden DA. Modern food microbiology. New York: Springer; 2005. 790 p. https://doi.org/10.1007/b100840
- Birhanu T, Mezgebu E, Ejeta E, Gizachew A, Nekemte E. Review on diagnostic techniques of bovine tuberculosis in Ethiopia. Report and Opinion. 2015;7(1):7–14.
- Verma AK, Tiwari R, Chakraborty S, Neha, Saminathan M, Dhama K, et al. Insights into bovine tuberculosis (bTB), various approaches for its diagnosis, control and its public health concerns: An update. Asian Journal of Animal and Veterinary Advances. 2014;9(6):323–344. https://doi.org/10.3923/ajava.2014.323.344
- Lema AG, Dame IE. Bovine tuberculosis remains a major public health concern: A review. Austin Journal of Veterinary Science and Animal Husbandry. 2022;9(1).
- Firdessa R, Tschopp R, Wubete A, Sombo M, Hailu E, Erenso G, et al. High prevalence of bovine tuberculosis in dairy cattle in central Ethiopia: Implications for the dairy industry and public health. PLoS ONE. 2012;7(12). https://doi.org/10.1371/journal.pone.0052851
- Cadmus SIB, Yakubu MK, Magaji AA, Jenkins AO, van Soolingen D. Mycobacterium bovis, but also M. africanum present in raw milk of pastoral cattle in north-central Nigeria. Tropical Animal Health and Production. 2010;42:1047–1048. https://doi.org/10.1007/s11250-010-9533-2
- Supply P, Mazars E, Lesjean S, Vincent V, Gicquel B, Locht C. Variable human minisatellite‐like regions in the Mycobacterium tuberculosis genome. Molecular Microbiology. 2000;36(3):762–771. https://doi.org/10.1046/j.1365-2958.2000.01905.x
- Cousins DV. Mycobacterium bovis infection and control in domestic livestock. Revue Scientifique et Technique. 2001;20(1):71–85. https://doi.org/10.20506/rst.20.1.1263
- Anaelom NJ, Ikechukwu OJ, Sunday EW, Nnaemeka UC. Zoonotic tuberculosis: A review of epidemiology, clinical presentation, prevention and control. Journal of Public Health and Epidemiology. 2010;2(6):118–124.