Аннотация
As a result of the analysis of the technological system for the production of dry granulated food concentrates, it has been revealed that the key contradictions in the formation of product quality arise in the subsystem of concentration of an extract and whey mixture. In the present paper on the improvement of the technology of instant granular products, including those on the basis of a chokeberry extract and milk whey, the process of concentration of their mixture has been studied, and the quality of the instant granular breakfast prepared using a concentrate has been estimated. To solve technical contradictions, studies were carried out using an example of the chokeberry extract, obtained both from the dried and frozen raw materials subjected to grinding in a mixture with curd whey. The combined concentration of whey and the extract made it possible to avoid the foaming characteristic of whey concentration, and also to exclude the process of mixing high viscosity masses from the technological flow, and the replacement of the processes of "drying and grinding" with "freezing and grinding" made it possible, first, to intensify the process and, second, to ensure a more complete extraction of BAS. A mathematical model of the process has been created, the regimes and parameters of concentration have been developed in a rotary-film evaporator operating under vacuum; the regulated indicators of nutritional value, the terms and modes of storage have been determined.Ключевые слова
Concentrating, extract, chokeberry, whey, concentration process, process model, quality indicatorsВВЕДЕНИЕ
The indicators of the quality of a technological flow, such as reliability, controllability, efficiency, product quality, etc., are interrelated and interdependent and condition each other. The improvement of some indicators often causes the deterioration of others. The analysis of the bottlenecks in the technological system aims at finding the key contradictions. To find the key contradiction and reveal its essence is half the success when creating a new technology [4, 5]. It should be borne in mind that the knowledge of regularities, limited by a process in a machine or apparatus, without taking into account the regularities characteristic of the system as a whole, will not lead to an optimal solution of a problem. To resolve technical contradictions effectively, a theoretical and experimental study of all processes and their subsequent optimization are required to reduce the sensitivity of a particular process in the system to the fluctuations in the input parameters [4].
ОБЪЕКТЫ И МЕТОДЫ ИССЛЕДОВАНИЯ
In the present paper on the improvement of the technology of instant granular products [6, 7, 8], including those on the basis of a chokeberry [10, 11, 19, 21, 22] and whey [21, 23] extract, the process of concentration of their mixture has been studied and the quality of the instant granular breakfast prepared using a concentrate has been estimated. The combined concentration of whey and the extract made it possible to avoid the foaming characteristic of whey concentration, and also to exclude the process of mixing high viscosity masses from the technological flow, and the replacement of the processes of "drying and grinding" with "freezing and grinding" made it possible, first, to intensify the process and, second, to ensure a more complete extraction of BAS.
The process of concentrating a chokeberry extract together with curd whey (with a ratio of 3 : 1) to 55–60% of solids is carried out using a vacuum rotary-film evaporator at a temperature of 48–50°C, which allows us to preserve thermolabile substances, due to this, the derived semifinished product in the form of a concentrate has both chemical and microbiological stability.
Of particular importance, from the point of view of forming the quality of the finished products, is the process of concentrating an extract and whey mixture [13, 14, 15]. The mathematical model of an evaporation process in a rotary-film evaporator has been constructed and the modes of concentrating extracts have been studied.
To study the intensification of the process of concentrating the mixture of chokeberry and curd whey extracts, a vacuum rotor-film evaporator was designed and manufactured.
A feature that distinguishes this device from similar devices is the evolved evaporation surface regulated by a set of disk elements installed on the rotor shaft, which are film formers [16, 19] and an adjustable shaft speed.
The initial solution in the evaporator is a mixture of whey and extract, is fed through the inlet to the maximum filling of the apparatus, limited by the contact of the liquid with the lower edge of the disc shaft. This requirement is caused by the fact that the efficiency of the apparatus depends on the area of the evaporation surface, and the wetting of the discs, the area of the film and the uniform distribution depend on the area of contact between the disc and the liquid when the rotor moves. The heat transfer processes in vacuum provide the evaporation and concentration of a solution.
In the heat-exchange rotor-film apparatus of the developed design, there is no contact between the heat transfer medium and the process medium during heat exchange, the heat is transferred through a transfer wall.
The operation of a rotary-film evaporator depends on a variety of factors [12, 13, 14]: the conditions of the process; the geometric dimensions of plates; the thickness of the film layer of the solution; the rotor design; the properties of the process medium; the rotor speed. When designing any technical system, ours is no exception; the main task is to provide the maximum intensity of the process with the least (optimal) energy costs.
In our case, this is the calculation of the main characteristics of a rotary-film evaporator when concentrating a mixture of curd whey with a chokeberry extract. In this regard, it seems reasonable to consider the heat-mass transfer and hydrodynamic processes that occur inside a vacuum rotor-film evaporator.
РЕЗУЛЬТАТЫ И ИХ ОБСУЖДЕНИЕ
The liquid film formed by self-wetting on the surface of the plate will perform a rotational motion therewith. At the same time, internal and external forces act on each film particle, the main ones of which are: gravity; the centrifugal force; adhesion to the surface of the disc and the force of the surface tension of the liquid.
The centrifugal force depends on a rotor speed, disc radius and film weight:
The given mass of the film is determined as the product of the thickness by the area occupied thereby on the surface of the disk by the density:
The calculations show that the centrifugal force is low, with the surface area of the disc in our apparatus S = 10–2 m2, the film thickness, according to an approximate calculation, b = 10–5 m, and the liquid density within p = (0.9–1.0)103 kg/m3, (the mass in this case is approximately equal to 10–4 kg), it can also be neglected.
The gravity of the film spread on the surface of the disk can also be neglected, due to its low thickness. Moreover, this thickness changes increasingly when the solution is concentrated and corresponding to an increase in viscosity. The liquid film is retained on the surface of the disk by the adhesion forces and does not have its own motion, and the possible displacement of part of it relative to the disk surface during one rotation under the action of the above forces will be insignificant and depends on the emerging internal shear forces that obey Newton's law. In this case, the force of the surface tension of the liquid caused by the intermolecular interaction inside the liquid is proportional to the dynamic viscosity of the solution and the shear rate gradient over the layer thickness, and the force of adhesion with the surface of the disk exerts resistance to the rupture of the film and its shear.
The heat-mass transfer, under such conditions when the hydrodynamic component is insignificant and close to zero in calculations, is simplified, since most of the similarity criteria describing this process are based on a velocity gradient.
Thus, based on the above calculated parameters characterizing the process, we can introduce a number of assumptions:
– the liquid film formed by adhesion on the surface of the rotor disk has a constant thickness and the relative shear of its layers is close to zero;
– the process temperature does not change with time and the heat exchange regime is steady inside the device;
– the heat flow is constant in the apparatus chamber;
– the losses of heat for concentration and convection during thermal calculations is included in the total value of the heat flow.
As can be seen from the graph of dependence of the evaporation area on the height of the liquid level presented in Fig. 1, with a decrease in the liquid level in the apparatus, it increases nonlinearly, as it was expected.
Assuming that the rotor speed is n (s-1), we can calculate the initial mass flow rate using the following formula:
The efficiency of the evaporator for the secondary steam can be determined in this case using the formula:
The efficiency of the vacuum rotor-film evaporator for the secondary steam, depending on equation (4), depends on the final concentration of the evaporated liquid, which cannot exceed 100% and must be known in advance, in accordance with the process conditions. In this case, the amount of heat supplied to the evaporated liquid is used to heat it from the initial temperature to the boiling point in a given vacuum and, in fact, for evaporation. In accordance with these assumptions, the heat flow in the apparatus can be determined using Fourier's law.
Thus, the most important parameter (along with others) was found – the rotor speed, which can vary within a certain range. Substituting GH from formula (3) and W from equation (4) into the heat balance equation and expressing n from it, we obtain the following relationship:
(1) The efficiency of the evaporator in accordance with equations (3) and (4) can continuously increase depending on an increase in the rotor speed and a decrease in the liquid level; herewith, the area of the evaporation surface increases.
(2) The amount of heat, and consequently the amount of energy consumed in accordance with the heat balance equation, increases.
If Item 1 fully corresponds to the solution of the problem of intensifying the concentration process in a vacuum rotary-film evaporator, the consideration of Item 2 showed that a continuous increase in the efficiency of the device due to an increase in the rotor speed while maintaining the overall dimensions leads to the fact that the amount of the heat required cannot be provided in full. Hence the need for the optimal use of thermal energy (heat flow), which provides a technological process. Thus, it was necessary to introduce a fourth assumption on the constancy of the heat flow in the evaporator. Therefore, in this case Q will not depend on an increase in the rotor speed n.
In the proposed device of the rotary-film evaporator, the device is heated by the thermal envelope, and consequently, a wall of the body of a given thickness, the area of which can be determined on the basis of the geometric dimensions of the evaporator under study, will be used as the heat transfer surface:
The main characteristics of the proposed apparatus can be calculated theoretically on the basis of the accepted mathematical relationships which are known or obtained on the basis of the known ones [16, 19].
Based on the above equations, the block diagram of the algorithm for calculating the main design parameters was developed (Fig. 2).
Table 1 presents the initial and calculated parameters.
The mathematical model of the developed vacuum rotor-film evaporator was constructed on the basis of which a method for calculating its basic characteristics was developed, taking into account the operating features of the apparatus, the conditions of the technological mode for processing solutions due to their specific properties.
Fig. 3 presents a graph of a change in the efficiency of the rotary evaporator depending on the liquid level in the apparatus.
An important role in calculating the main characteristics is played by the rotational speed of the rotor shaft of the evaporator. In this case, the heat flow is independent of the rotational speed of the shaft and remains constant.
The initial mass flow (the efficiency for the initial solution) GH, calculated using the formula (3), will increase in direct proportion to an increase in the rotor speed. This is due to the fact that more liquid will be required for processing to renew a film layer at a higher rotation speed.
When calculating the rotor speed using formula (5), it can be noted that there is only one value of n for a particular process model the parameters of which must be fixed. In this case, the only variable (within small limits) is the value of XK (the final concentration of the processed extract).
After tabulating the values of XK, using formula (5), the rotor speed was calculated. Table 1 presents the values of the rest of the parameters of the experimental unit. Fig. 4 shows the obtained (inverse) relationship.
As can be seen from Fig. 4, with an increase in the rotor speed, the final concentration of the processed product (other things being equal to the process) drops sharply.
The solution concentration cannot be higher than 100% and lower than the initial value of XH, [14, 19]. Substituting these values as boundary conditions in equation (5), we obtain the maximum and minimum rotational speed:
when XK = XH
The points obtained from formulas (7) and (8) are indicated on the X axis as the minimum and maximum values of the rotational speed n. In this case, the X axis was divided into several intervals.
Fig. 5 shows a change in the dominant characteristics of the vacuum rotor-film evaporator depending on the rotor speed.
Considering the "behavior" of the curves in each of the characteristic segments, it can be noted that
(1) In the interval of a change in the rotation speed from 0 to nmin, the efficiency of the evaporator increases by the amount of evaporated liquid; and the final concentration of the solution reaches 100%. The amount of heat supplied will not be completely absorbed by an evaporation process, which will result in the additional heating of the final product, which is unacceptable for the processing of the liquids containing biologically active or thermolabile substances. The operation of the device in this range will result in the unreasonable consumption of energy and loss of quality of the final product. In this case, the speed value equal tonmin, which can be calculated using formula (8), will be optimal for obtaining a product with the maximum concentration under the given process conditions.
(2) In the interval from nmin to nmax, the efficiency for the secondary steam will increase for some time, and then, approaching the speed nmax, smoothly decrease, tending to a certain minimum value. In this interval, a point is fixed that corresponds to such a value of the rotor speed at which the efficiency of the evaporator for the secondary steam corresponds to the maximum value. The concentration of the resulting solution will not reach the maximum value.
(3) With a further increase in the number of rotations of more than nmax, the efficiency for the secondary steam remains practically unchanged. Since the amount of heat is constant, this amount will not be enough to intensify the process of evaporation. In this case, the heat is only consumed to heat the liquid supplied to the evaporator. Then, in this interval, the device will operate as a heat exchanger and it will be inappropriate to use it to concentrate liquids.
Thus, one of the main optimization parameters for the design and operation of devices based on a vacuum rotary-film evaporator was set: is the rotational speed of the rotor n, which can be calculated from equation (5).
By transforming the obtained data, it is possible to calculate an optimal method for obtaining a mixture of curd whey extracts and a chokeberry extract using a special program for the given geometric dimensions and process conditions, determining in advance the efficiency of the rotary evaporator. Table 2 shows the initial data for this calculation and the optimal operating parameters of the rotary-film evaporator.
The discrepancy between the experimental data and the calculated ones for the reference liquid (water) did not exceed 10% with allowable deviations for mathematical models up to 30%. Hence it can be concluded that the calculated values of the main parameters of the rotary evaporator for extracts will correspond to the real ones with an accuracy of 10%.
At the same time, attention is drawn to the fact that, under equal experimental conditions, the fixed parameters of the process using an experimental sample of the apparatus are as follows: with an increase in the evaporation area by a factor of 10, the efficiency of the evaporator for the secondary steam increased by 6 times with the same thermal load of the device.
Such a significant increase in the coefficient of utilization of the thermal energy supplied to the evaporator is a sign of its high efficiency; while the energy costs in such devices are insignificant in comparison with the achieved effect of process intensification.
Taking into account the changes in the subsystem in obtaining a concentrated mixture of extract and whey [23–26], a new technology of instant granular breakfast was proposed.
Fig. 6 presents the technological scheme of production of the mixtures being developed.
After inspection, the fruit and berry raw materials are dried at a temperature of 50–55°C, which allows us to preserve thermolabile substances or frozen at a temperature of -22 degrees Celsius.
Then the fruits are ground to a size of 0.5–1.5 mm [25], which allows us to achieve the necessary phase contact of the surfaces between the extractant and the plant mass, which allows us to significantly reduce the hydrodynamic resistance, especially when using aqueous solvents, when the swelling of plant raw materials is possible.
The ground fruit and berry raw materials are extracted. At the same time, the raw materials / extractant ratio for the dried raw materials is 1/6–1/7; for the frozen raw materials – 1/8–1/10 at an extraction temperature of 40–50°C.
Water, ethanol or their solutions are used in different concentrations as solvents. Varying the extractants and the characteristics and conditions of the process allows us to change the spectrum of the extracted substances, as well as to fractionate them. Applying them in combinatorics or sequentially, it is possible to achieve the complete extraction of extractive substances from the raw materials. In this case, the extracts obtained have not only a different biological activity, but also a completely different type of action. The raw materials that have the same groups of high-molecular substances and the same structural and mechanical properties in their composition, can be extracted together [17, 19, 22].
After extraction, the resulting mixture is filtered and the extract is separated from the meal. The latter is dried to a humidity of 8–10% and ground to a particle size of 100–200 microns.
At the next step, the powdered sugar is premixed in a 3 : 1 ratio with dry cheese whey and is supplied into a mixer where the resulting mixture and the remaining dry and liquid ingredients that form part of the breakfast formulation are mixed. This order of feeding ingredients into the mixer allows them to be spread uniformly and, as a result, to obtain a more homogeneous breakfast mixture.
The prepared polydisperse multicomponent mixture moistened to 8–10% is fed into a pelletizer with a dispersant. The dispersant provides the homogeneity of granules, and also increases efficiency due to more intensive nucleation. In the process of granulation, the amount of the liquid phase is corrected to the optimum by spraying the concentrated mixture of the liquid constituent of the formulation through a nozzle.
The resulting breakfast granules are subjected to convective drying at a temperature of 50–55°C to a humidity of 8–9%.
The dried granulate is fed to a vibrating screen, where it is sorted by size: 1–3 mm ones are fed into the hopper; the ones that have a size of less than 1 mm and more than 3 mm are fed for further processing or grinding.
The finished product – "dry instant granular whey-based breakfast" is sent for packing and packaging.
From a physico-chemical point of view, the technology for the production of instant granular products suggests the creation of systems with a certain phase composition and structure (the presence of capillaries throughout the cross section of granules). Therefore, the granulation process requires the presence of an automatic process control system, and therefore, a permanent control over the concentrations of liquid, solid and gaseous phases at all stages of the formation of a porous system (mixing, coagulation, condensation, crystallization).
The analysis of the performed diagnostics of granulation processes made it possible to establish that the law of constancy of the volume phase composition of a polydisperse system makes it possible to graphically depict the process of structure formation at any technological stage in the triple Ks-Ke-Kg system of coordinates. The resulting phase diagram of the entire technological process is a phase portrait of a technology.
The use of the phase diagrams based on the law of constancy of the volume phase composition of disperse systems gives the opportunity to conduct both the theoretical and practical analysis of various technologies, technological stages and operations with the aim of obtaining materials with specified properties. In addition, it makes it possible to improve the effectiveness of technological control in the production of materials on the basis of disperse systems, to simplify the methods for their study, and also allows the introduction of standardization elements in scientific research.
The fractional composition of the product has been studied. It has been revealed that most of the granules of both samples are 1.5–2.0 mm in size, which agrees well with the law of normal distribution, and confirms our assumptions about the correct choice of the technological parameters of the granulation process, as well as the estimation of their effect on the quality of granules. The shape of this curve characterizes the monodispersity of the obtained granulate.
The capillary-porous structure of granular products significantly affects the properties of the finished product. To characterize the porosity, we used the porosity index, which is determined by the ratio of the volume of the water that penetrates into the granule of the dry product and the volume of the granule:
Fig. 7 shows the phase diagrams that characterize the changes in the ratio of the solid, gas and liquid components in the technology of granulating a chokeberry-based product with the addition of whey.
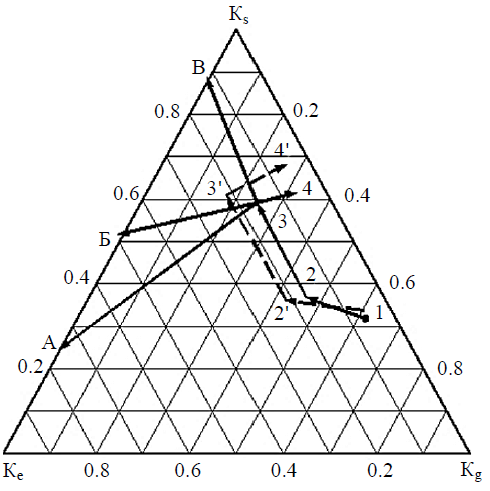
Table 3 presents the formulation of the developed product.
The physicochemical, microbiological and organoleptic studies of the quality and safety indicators of the developed product in the production and storage process have been carried out.
The technological modes and parameters for the production of dry instant granular breakfast allowed us to determine the regulated quality indicators, including a nutritional value (Table 4).
Breakfast is packed in the containers allowed for a contact with food products that excludes a contact with moist air. The product should be stored at a temperature of 20 ± 2°С and a relative humidity of 75 ± 5%.
ВЫВОДЫ
Based on the study of the dynamics of organoleptic, microbiological and physico-chemicalproperties in the process of production and storage, the regulated indicators of the quality of the instant breakfast based on the extract of chokeberry and curd whey have been established. The obtained results have become the basis for establishing a shelf life – 6 months from the date of manufacture.
The developed breakfast meets the requirements for specialized products with directional functional properties in composition, properties and a nutritional value, and can be a reliable means of correcting the deficiency of vitamins, minerals and the related metabolic processes [20].
The ready-to-drink beverage is prepared by dissolving 25 g of instant granular breakfast in 200 ml of hot (85–95°C) water. This volume of the beverage provides the necessary preventive amount of the adult's need for essential nutrients, taking into account their content in the traditional daily diet.
Technical documentation has been developed and approved; the industrial production is carried out at Scientific and Production Association “Healthy Nutrition” [“Zdorovoe pitanie”] (Kemerovo, Russia).
СПИСОК ЛИТЕРАТУРЫ
- Antipov S.T., Panfilov V.A., Urakov O.A., and Shakhov S.V. Sistemnoe razvitie tekhniki pishchevykh tekhnologiy [System development of food technology]. Moscow: Kolos Publ., 2010. 762 p.
- Panfilov V.A. (ed.). Teoreticheskie osnovy pishchevykh tekhnologiy [Theoretical foundations of food technology]. Moscow: Kolos Publ., 2009. 1408 p.
- Popov A.M., Tikhonov V.V., Tikhonov N.V., Tikhonova I.N., Donya D.V., and Miller E.S. Sposob polucheniya instant-produktov na osnove kontsentratov plodovo-yagodnykh sokov, soderzhashchikh razlichnye funktsional'nye dobavki [A method of obtaining instant products on the basis of concentratedfruit-and-berry juice containing various functional additives]. Patent RF, no. 2608729, 2017.
- Tikhonova I.N., Popov A.M., Tikhonov N.V., and Tikhonov V.V. Harnessing the Capabilities of Spray Granulation in the Food Industry for the Production of Functional Foods. Procedia Chemistry, 2014, vol. 10, pp. 419–423. DOI: 10.1016/j.proche.2014.10.070.
- Jabri Karoui I. and Marzouk B. Characterization of bioactive compounds in Tunisian bitter orange (Citrus aurantium L.) peel and juice and determination of their antioxidant activities. BioMed Research International, 2013, vol. 2013, no. 3454415, pp. 1–12. DOI: 10.1155/2013/345415.
- Jakobek L., Šeruga M., and Krivak P. The influence of interactions among phenolic compounds on the antiradical activity of chokeberries (Aronia melanocarpa). International Journal of Food Sciences and Nutrition, 2011, vol. 62, no. 4, pp. 345–352. DOI: 10.3109/09637486.2010.534438.
- Kulling S.E. and Rawel H.M. Chokeberry (Aronia melanocarpa) – A review on the characteristic components and potential health effects. PlantaMedica, 2008, vol. 74, no. 13, pp. 1625–1634. DOI: 10.1055/s-0028-1088306.
- Plyatsuk L.D., Shaporev V.P., Moiseev V.F., et al. Osnovy gidrodinamiki i massoperenosa v rotornykh massoobmennykh apparatakh [Fundamentals of hydrodynamics and mass transfer in rotary mass-exchange machines]. Sumy: SSU Publ., 2013. 179 p.
- Popov A.M. and Litvina E.A. Technology of production of granulated chokeberry jelly based on whey. Storage and processing of farm materials, 2001, no. 1, pp. 39–41. (In Russian).
- Kravchenko S.N., Pavlov S.S., and Popov A.M. Production of fruit and berry extracts of various forms and functional purposes. Beer and beverages, 2005, no. 3, pp. 38–41. (In Russian).
- Maytakov A.L. Teoreticheskie osnovy obespecheniya kachestva funktsional'nykh elementov pishchevykh mashin na osnove formirovaniya modeley tekhnologicheskikh blokov [Theoretical bases of maintenance of quality of functional elements of food machines on the basis of formation of models of technological blocks]. Kemerovo: Kuzbassvuzizdat Publ., 2010. 139 p.
- Ivanov P.P. Razrabotka tekhnologii i apparaturnogo oformleniya proizvodstva kontsentrirovannykh plodovoyagodnykh ekstraktov dlya molochnoy promyshlennosti [Development of technology and hardware registrationof production of the concentrated fruit and berry extracts for the dairy industry]. Diss. Cand. Sci. (Eng.). Kemerovo, 2002. 134 p.
- Khramtsov A.G. and Nesterenko P.G. Tekhnologiya produktov iz molochnoy syvorotki [Technology of whey products]. Moscow: Deli Print Publ., 2003. 768 p.
- Wu X., Beecher G.R., Holden J.M., et al. Concentrations of anthocyanins in common foods in the United States and estimation of normal consumption. Journal of Agricultural and Food Chemistry,2006, vol. 54, no. 11, pp. 4069–4075. DOI: 10.1021/jf060300l.
- Keshani S., Luqman C.A., Nourouzi M.M., et al. Optimization of concentration process on pomelo fruit juice using response surface methodology (RSM). International Food Research Journal, 2010, vol. 17, no. 3, pp. 733–742.
- Maskan M. Production of pomegranate (Punica granatum L.) juice concentrates by various heating methods: Colour degradation and kinetics. Journal of Food Engineering, 2006, vol. 72, no. 3, pp. 218–224. DOI: 10.1016/j.jfoodeng.2004.11.012.
- Olevskiy V.M. Rotorno-plenochnye teplo- i massoobmennye apparaty [Film heat and mass transfer equipment]. Moscow: Khimiya Publ., 1988. 240 p.
- Jurgonski A., Juskiewicz J., and Zdunczyk Z. Ingestion of black chokeberry fruit extract leads to intestinal and systemic changes in a rat model of prediabetes and hyperlipidemia. Plant Foods for Human Nutrition, 2008, vol. 63, no. 4, pp. 176–182. DOI: 10.1007/s11130-008-0087-7.
- Valcheva-Kuzmanova S., Borisova P., Galunska B., et al. Hepatoprotective effect of the natural fruit juice from Aronia melanocarpa on carbon tetrachloride-induced acute liver damage in rats. Experimental and Toxicologic Pathology, 2004, vol. 56, no. 3, pp. 195–201. DOI: 10.1016/j.etp.2004.04.012.
- Zologina V.G. Tekhnologiya kompleksnoy pererabotki plodov ryabiny obyknovennoy [Technology of complex processing of mountain ash fruits]. Abstract of Diss. Cand. Sci. (Eng.). Krasnoyarsk, 2005. n. p.
- Novoselov S.V. Metodologiya proektirovaniya i prodvizheniya na potrebitel'skiy rynok pishchevykh produktov v usloviyakh innovatsionnoy deyatel'nosti [Methodology of design and promotion on the market of new food products in the context of innovation activity]. Diss. Dr. Sci. (Eng.). Kemerovo, 2012. 385 p.
- Spirichev V.B. (ed.), Shatnyuk L.N., and Poznyakovskiy V.M. Obogashchenie pishchevykh produktov vitaminami i mineral'nymi veshchestvami. Nauka i tekhnologiya [Enrichment of foods with vitamins and minerals. Science and technology]. 2nd ed. Novosibirsk: Sib. Univ. Publ., 2005. 548 p.
- Vagiri M. and Jensen M. Influence of juice processing factors on quality of black chokeberry pomace as a future resource for colour extraction. Food Chemistry, 2017, vol. 217, pp. 409–417. DOI: 10.1016/j.foodchem.2016.08.121.