Аннотация
Punica granatum L. possesses significant nutritional and medicinal potential. Its pharmacological activities have been investigated, but no comparative evaluation has been reported regarding the effect of different extraction solvents on the phytochemical content and antioxidant activity of its leaf, bud, and flower extracts.This research involved seven various solvents, namely methanol, ethanol, water, acidified methanol, acidified ethanol, acidified water, and hexane. A set of experiments made it possible to define the effect of each of these solvents on the contents of phenolics, flavonoids, flavanols, flavonols, anthocyanins, and tannins, as well as on the antioxidant activity of pomegranate leaf, bud and flower tissues. The research objective was to identify the optimal solvent for the most effective extraction of the abovementioned functional compounds. The antioxidant activity tests involved DPPH free radical scavenging, metal chelating, iron (III) reducing power, and CUPRAC assays.
The aqueous extract of P. granatum leaves demonstrated the highest total phenolic content (192.57 mg GAE/g extract) while the greatest flavonoid content belonged to the acidified methanol extract of P. granatum buds (73.93 mg RE/g extract). The HPLC analysis detected such significant phenolic compounds as punicalagin in buds and flowers, as well as gallic acid in leaves. All the extracts showed good antioxidant activity; however, the bud extracts had a better antioxidant profile than the extracts from leaves and flowers.
The pomegranate leaf, bud, and flower extracts demonstrated excellent phytochemical and antioxidant properties, which makes it possible to recommend these plant tissues as raw materials to be used in pharmaceutical, food, nutraceutical, and cosmetic industries.
Ключевые слова
Punica granatum L., pomegranate buds, pomegranate leaves, pomegranate flowers, antioxidant activity, phytochemical analysis, pharmacological potentialВВЕДЕНИЕ
Reactive oxygen species, such as superoxide anion (O2-), hydrogen peroxide (H2O2) and hydroxyl radical (HO·), comprise both free radical and non-free radical species formed by the partial reduction of oxygen [1, 2]. Reactive oxygen species are a result of mitochondrial respiration. It involves a variety of enzymes, e.g., nicotinamide adenine dinucleotide phosphate oxidase, xanthine oxidase, nitric oxide synthase, and peroxisomal constituents [3]. However, environmental toxins, ultraviolet and ionizing radiation, quinone compounds, inflammatory cytokines, tobacco smoke, and some pharmaceutical agents can also trigger reactive oxygen species production [3, 4].
Antioxidants and their functions in physiological cell processes can normally balance reactive oxygen species. At high concentrations, reactive oxygen species become pathological and cause oxidative stress [2–5]. Oxidative stress is defined as an imbalance between oxidants and antioxidants caused by excessive production of reactive oxygen species [3, 6]. Oxidative stress is responsible for such cardiovascular diseases as atherosclerosis, ischemia, hypertension, and cardiomyopathy. It is known to cause cancer, kidney diseases, food allergies, and other allergic conditions, e.g., asthma, rhinitis, atopic dermatitis, etc. Oxidative stress is also associated with such neurological diseases as depression, memory loss, amyotrophic lateral sclerosis, Parkinson disease, Alzheimer disease, etc. [7, 8]. Natural antioxidants maintain the delicate balance between oxidants and antioxidants, thus protecting the cell from the harmful effect of reactive oxygen species [8].
Synthetic antioxidants include butylated hydroxyanisole, butylated hydroxytoluene, propyl gallate, and tert-butyl hydroquinone. They can also be used to avoid the harmful effects of oxidative stress. However, a longterm intake has been found to increase the risk of cancer [9, 20]. Herbs, species, seeds, fruits, vegetables, and edible mushrooms are natural sources of antioxidants [11].
The pomegranate (Punica granatum L.) is one of the world’s oldest cultivated and consumed fruit [12]. This plant belongs to the Lythraceae family [13]. Pomegranates are cultivated in Turkey, Morocco, Spain, Afghanistan, Egypt, Italy, India, China, Pakistan, Africa, Israel, Japan, the USA, Russia, Australia, and Saudi Arabia [14]. Pomegranates are valued for their peel, seeds, arils, flowers, and leaves. They are an important source of phytochemicals with proven antioxidant properties [15–20]. Different parts of pomegranate cure a variety of diseases in Islamic, Ayurvedic, Persian, Unani, and Chinese folk medicines. Pomegranate bark and root treat diarrhea, dysentery, and ulcers; seeds are used to treat anorexia and dyspepsia; flowers are applied in case of gingival inflammation, gomphosis, and other dental ailments [21]. In addition, pomegranate extracts demonstrate important pharmacological activities. Leaf extracts exhibit anticancer, antibacterial, anti-diabetic, anti-inflammatory, anticholinesterase, and antigenotoxic effects [19, 22–25]. Flower extracts demonstrate anti-diabetic, anti-inflammatory, analgesic, antibacterial, hepatoprotective, and antispasmodic properties [26–31]. Bud extracts show antihistaminic and antiproliferative activities [32, 33].
The pharmacological properties of pomegranate leaf, flower, and bud extracts attract scientific attention due to their antioxidant activity and phytochemical content. In plants, phytochemical components and antioxidant properties depend on such factors as genotype, climate, maturity, cultivation practice, region, and extraction solvent [34–36]. The extraction solvent is a significant factor that affects both quality and quantity [37]. Wakeel et al. demonstrated the importance of solvent type and polarity [38]. In their study of Isatis tinctoria L., methanol-ethyl acetate proved to be the most efficient solvent in terms of total phenolic content in leaves while methanol and acetone-water were the most effective solvents in flower extracts. Elfalleh et al., who studied P. granatum flowers, leaves, seeds, and peels, reported methanol to be more effective solvent for polyphenols than water [39].
Therefore, each plant has its own most effective extraction solvent. According to the literature that we reviewed, pomegranate buds are low in antioxidant activity. This research featured the effect of seven different extraction solvents on the phytochemical content and antioxidant activity of pomegranate leaves, flowers, and buds. We also investigated the relationship between their phytochemical content and antioxidant activity.
ОБЪЕКТЫ И МЕТОДЫ ИССЛЕДОВАНИЯ
Chemicals and equipment. The research included trichloroacetic acid (TCA), ethylenediaminetetraacetic acid (EDTA), butylated hydroxytoluene (BHT), butylated hydroxyanisole (BHA), 1,1ʹ-diphenyl-2-picryl hydrazyl (DPPH), α-tocopherol (α-TOC), ferrous chloride, sodium carbonate, sodium acetate, aluminum chloride, 4-(dimethyamino)cinnamaldehyde (DMAC), and neocuprine. These chemicals were purchased from Sigma, USA. Ethanol, methanol, glacial acetic acid, ferric chloride, ammonium acetate, copper (II) chloride, and formic acid came from Merck, Germany. Gallic acid and n-hexane were purchased from Riedel-de Haën. All other reagents and solvents were of analytical grade. The research involved such equipment as a lyophilizer (Christ Alpha 1–2 LD Plus), a heating mantle (Daihan WHM 12013), a rotary evaporator (Laborota 4000-efficient Heidolph), a spectrophotometer (Shimadzu UV-1601), a shaking water bath (Clifton 100–400 rpm), a centrifuge (Nüvefuge CN180), a vortex (Fisons), a pH meter (Jenco 6177), and a high-performance liquid chromatographer (Shimadzu) with a diode array detector (DAD).
Plant materials. Buds, flowers, and leaves of Punica granatum L. were picked up manually in Adıyaman (Kâhta, southeast territory of Anatolia) in 2020. A voucher specimen (ZT1001) was identified by Professor Turan Arabacı (İnönü University). It was stored at the herbarium of the Pharmacy Department of İnönü University, Malatya. Each of the samples was dried in a freeze dryer for two days and then pulverized.
Preparing P. granatum extracts. We extracted 20.0 g of each sample, i.e., leaves, flowers, and buds, with different solvents in the following order: methanol, ethanol, water, n-hexane, 1.0% formic acid in methanol, 1.0% formic acid in ethanol, and 1.0% formic acid in water. The procedure involved a Soxhlet extractor and lasted for 4 h. The extract was concentrated at 40°C in a rotary evaporator. All the extracts were kept in an airtight container and stored at 4°C in a refrigerator.
Determining total phenolic content. We used the Folin-Ciocalteu assay with slight modifications to estimate the total phenolic content in all extracts [40]. In brief, 50 μL of extract solutions were mixed with 450 μL of distilled water and 250 μL of 10-fold diluted 2.0 N Folin-Ciocalteu reagent. We vortexed the mix and incubated it for 5 min before adding 2500 μL of 2.0% Na2CO3 and vortexing it once more. After incubating, all the samples remained at room temperature for 2 h. After that, their absorbance was measured at 765 nm using an ultraviolet-visible spectrophotometer. The calibration curve was constituted using gallic acid (GA). Finally, we determined the total phenolic content using the calibration line of gallic acid and expressed it as mg GAE/g extract, i.e., 1 mg gallic acid equivalent per 1 g of extract.
Determining total flavonoid content. We used the aluminum chloride colorimetric method to define the total flavonoid content in all extracts [41]. We incubated a mix of 500 μL of extract solution, 4500 μL distilled water, and 300 μL NaNO2 (1:20) at room temperature for 5 min before adding 300 μL of AlCl3 (1:10). After 6 min of incubation, we added 2000 μL of NaOH (1.0 M) and 2400 μL of distilled water and vortexed the resulting mix. Its absorbance was registered at 510 nm with a spectrophotometer. We sued a calibration curve for rutin to determine the total flavonoid content, which we expressed as mg RE/g extract, i.e., 1 mg of rutin equivalent (RE) per 1 g of extract.
Determining total flavonol content. The total flavonol content was determined as described by Kumaran & Karunakaran [42]. According to the procedure, we mixed 1000 μL extract solution with 1000 μL AlCl3 (1:50) and 3000 μL CH3COONa (1:20). After incubating the mix at room temperature for 30 min, we measured the absorbance at 440 nm using a spectrophotometer. The total flavonol content was calculated based on the quercetin calibration curve and expressed the results as mg QUE/g extract, i.e., 1 mg of quercetin equivalent (QUE) per 1 g extract.
Determining total flavanol content. This procedure involved the dimethylacetamide (DMAc) reagent, as recommended by McMurrough & McDowell [43]. In this method, the reaction mix comprised 1.0 mL of extract and 3.0 mL dimethylacetamide (1:100). We vortexed the mix and allowed it to settle for 20 min. The absorbance was registered at 640 nm using an ultraviolet-visible spectrophotometer. We used the catechin calibration curve and expressed the results as mg CE/g extract, i.e., 1 mg catechin equivalent per 1 g extract.
Determining total anthocyanin content. This experiment involved a spectrophotometer and the pH differential method [44]. We used two buffer systems: potassium chloride buffer with pH of 1.0 (25.0 mM) and sodium acetate buffer with pH of 4.5 (0.4 M). We mixed 0.4 mL extract solution with 3.6 mL of each buffer and incubated the resulting mix at room temperature for 15 min. The absorbance was registered at 510 and 700 nm using an ultraviolet-isible spectrophotometer. The total anthocyanin amount was demonstrated as equivalents for cyanidin-3-glucoside (mg Cy3G/kg extract).
Determining total tannin content. We used the vanillin-HCl staining method as proposed by Broadhurst & Jones [45]. This method relies on the ability of tannin to react with vanillin in the presence of HCl, giving a pink-red color [46]. We added 1.0 mL of extract, 3.0 mL vanillin reagent (1:25), and 1.5 mL concentrated HCl to tubes wrapped in aluminum foil. After vortexing, we incubated the mix in the dark at room temperature for 15 min. The absorbance was registered at 500 nm. The outcomes were given as epicatechin equivalent (mg ECE/g extract).
HPLC analysis for individual phenolic compounds. To identify the phenolic composition of P. granatum extracts, we used a high-performance liquid chromatographer with a diode array detector. The chromatographic separation included an ACE column (5 μm, C18, 250×4.6 mm) heated at 30°C. Mobile phase A comprised 70.0% acetonitrile and 30.0% distilled water while mobile phase B comprised 2.0% acetic acid in water. The experimental conditions were as follows: 5:95 A: B for 0–3 min, 15:85 for 4–40 min, 70:30 for 41–50 min, 90:10 for 50.01–55 min, 95:5 for 56–60 min. The flow rate was 1 mL/min. The chromatograms were taken at 250, 280, 320, and 360 nm.
Determining DPPH free radical scavenging activity. The 2,2-diphenyl-1-picrylhydrazyl (DPPH) free radical activity was measured as described by Blois [47]. We took 3.0-mL extract solutions at concentrations of 12.5, 25.0, 37.5, 62.5, and 125.0 μg/mL and added 1.0 mL of DPPH solution. After vortexing the mix and incubating it in the dark at room temperature for 30 min, we measured the absorbance of the solution at 517 nm using an ultraviolet-visual spectrophotometer. We appealed to butylated hydroxytoluene (BHT), butylated hydroxyanisole (BHA), and α-tocopherol (α-TOC) as reference standards. The outcomes were given as percentage of DPPH radical scavenged by the extracts.
Determining ferrous ion chelating activity. We adopted the method described by Carter to specify the metal chelating activity [48]. In line with the procedure, we mixed the extract solutions at concentrations of 12.5, 25.0, 37.5, 62.5, and 125.0 μg/mL with 0.10 M sodium acetate buffer (pH 4.9) and 0.01% iron (II) chloride. After vortexing the mix and incubating it for 15 min, we added 40.0 mM ferrozine. After 10 min, we registered the absorbance at 562 nm, using ethylenediaminetetraacetic acid (EDTA) as positive control. The outcomes were given as percentage of inhibition of ferrozine-Fe2+ complex.
Determining reducing power. We used the method described by Oyaizu to measure the reducing power ability of P. granatum extracts [49]. We added 0.20 M phosphate buffer (pH 6.60) and 1.0% potassium ferricyanide to 1.0 mL extract solutions at the concentrations of 5.88, 14.70, 29.41, and 44.11 μg/mL. After vortexing, we incubated the resulting mix in a water bath at 50°C for 20 min, followed by centrifuging at 6000 rmp for 10 min. Subsequently, we transferred 1250 μL supernatants to another tube, adding 1250 μL of distilled water and 500 μL of iron (III) chloride. After vortexing, we registered the absorbance at 700 nm, using butylated hydroxytoluene (BHT), butylated hydroxyanisole (BHA), and α-tocopherol (α-TOC) as reference standards.
Determining total antioxidant capacity. This procedure involved the CUPRAC (cupric reducing antioxidant capacity) method as described by Apak et al. [50]. We added 1000 μL of 10.0 mM CuCl2, 1000 μL 7.5 mM neocuproine, 1000 μL 100.0 mM ammonium acetate buffer (pH 7.00), 100 μL extract solutions, and 1000 μL distilled water in the test tubes. After vortexing the mix and incubating it in the dark at room temperature for 30 min, we measured the absorbance at 450 nm using an ultraviolet-visible spectrophotometer. The outcomes were presented as μmol Trolox equivalents (μmol TRE/g extract).
Statistical analysis. All tests were carried out in triplicate. The results were given as mean ± standard deviations. We also performed the Tukey’s Honestly Significance Difference (HSD) test to compare significant differences between means.
РЕЗУЛЬТАТЫ И ИХ ОБСУЖДЕНИЕ
Pomegranate leaf, bud, and flower extract yield. Punica granatum L. leaves, buds, and flowers were extracted using solvents with different polarities. Acidified solvents were used to extract more phenolic compounds, depending on the nature of the polyphenols in the structure of the plant materials, characterized using the HPLC method. Acidified organic solvent was used to obtain phenolic extracts with high anthocyanin content. According to the yield results (Table 1), acidified methanol proved to be the most effective extraction solvent. However, the yields ranged from 0.91–40.75, 1.77–58.76, and 0.36–37.45% for leaf, bud, and flower extracts, respectively. The highest extraction yield was found in the bud extract.
In general, the bud extract registered the best yield in acidified methanol and methanol (58.76 ± 1.11 and 51.61 ± 1.59% respectively), followed by leaves (40.75 ± 0.46) and flowers (37.45 ± 0.64) in acidified methanol. Trabelsi et al. used six different solvents to extract P. granatum leaves: hexane, chloroform, ethyl acetate, ethanol, aqueous, and acetone/water [51]. The highest extract yield of 20.07% belonged to the aqueous leaves extract. In another study, Kaur et al. applied a Soxhlet extractor to flowers and obtained a 23.51% yield with 95.0% ethanol [52].
These differences in yield can be due to the method of extraction and the region where the plant came from. Likewise, the extraction yield depends on temperature, solvent, solvent/sample ratio, and extraction time, as well as on the particle size and chemical nature of plant tissues. Under the same extraction time and temperature, solvent and chemical composition remain the two most important factors [53, 54].
Total phenolic content in different pomegranate parts. To specify the total phenolic content of the leaf, bud, and flower extracts, we applied a regression equation of calibration curve (y = 0.0096x + 0.0742) and expressed the result as mg GAE/g extract, i.e., 1 mg gallic acid equivalent per 1 g of extract (Table 2). The amount of total phenolic extracted from leaves, buds, and flowers in different solvents ranged from 10.64 ± 0.07 to 192.57 ± 0.02 mg GAE/g extract. Among all the extracts, the aqueous extract of leaves had the highest phenolic content (192.57 ± 0.02 mg GAE/g extract), followed by the methanol and the acidified methanol extracts of buds (187.97 ± 0.03 and 187.16 ± 0.07 mg GAE/g extract), respectively.
According to our data, methanol proved to be the most effective solvent for extraction of phenolic compounds from pomegranate leaves, buds, and flowers. Also, the pomegranate bud extracts were rich in phenols. In our study, the total phenolic content in the leaf extract appeared to be higher than that reported by Bekir et al. and Elfalleh et al. [24, 39]. However, the phenolic content in the flower extract was lower than that reported by Zheng et al. [55]. In addition, the pomegranate bud extract in our study contained more total phenolics than that reported by Attanayake et al., which was 110.37 mg GAE/g [56]. Our results for total phenolic content were in agreement with those published by Orgil et al. who reported that pomegranate buds had more phenolics than leaves and flowers [33]. Such differences may be explained by the different extraction solvents and pomegranate cultivars.
Total flavonoid content in different pomegranate parts. To calculate the total flavonoid content in pomegranate leaf, bud, and flower extracts, we appealed to a regression equation of calibration curve (y = 0.005x + 0.0217) and expressed the obtained results as mg RE/g extract, i.e., 1 mg of rutin equivalent (RE) per 1 g of extract (Table 3). The amount of total flavonoid extracted from leaves, buds, and flowers in different solvents varied from 8.83 ± 0.29 to 73.93 ± 0.09 mg RE/g extract. Table 3 shows that the highest total flavonoid content (73.93 ± 0.09 mg of rutin in 1 g of extract) belonged to the acidified methanol bud extract whereas the lowest one (8.83 ± 0.29 mg rutin in 1 g of extract) belonged to the hexane leaf extract. Our results seem to be much higher than the total flavonoid content in the leaf and flower extracts recorded by Mekni et al. and Elfalleh [39, 57].
These differences result from the cultivar diversity. In addition, the acidified solution of methanol was more efficient than the other solvents used for flavonoid extraction. Kopjar et al. reported that acidified methanol extract had the highest total flavonoid content, which supports the results of our study [58].
Total flavonol content in different pomegranate parts. To specify the total flavonol content in the pomegranate leaf, bud, and flower extracts, we used a regression equation of calibration curve (y = 0.0148x + 0.0278) and expressed the results as mg QUE/g extract, i.e., 1 mg of quercetin equivalent (QUE) per 1 g extract (Table 4). In our case, the acidified methanol extract of pomegranate buds had higher flavonol content than the other extracts. The highest flavonol amount belonged to the pomegranate bud extracts.
Cheurfa et al. examined the antioxidant and antidiabetic properties of pomegranate leaves [19]. They reported the total flavonol contents in aqueous and hydroalcoholic extracts as 9.20 ± 2.80 and 7.68 ± 0.60 mg QUE/g extract, respectively. Although the abovementioned research gives an important amount of data about pomegranate, very little information is available regarding the total flavonol content of pomegranate leaves, buds, and flowers.
Total flavanol content in different pomegranate parts. To measure the total flavanol content in pomegranate leaf, bud, and flower extracts, we used a regression equation of calibration curve (y = 0.1317x + 0.0021) and expressed the obtained results as mg CE/g extract, i.e., 1 mg catechin equivalent per 1 g extract (Table 5). We detected some significant difference in flavanol content between extraction solvents and plant tissues. The amount of total flavanol extracted from leaves, buds, and flowers in different solvents varied from 0.12 ± 0.03 to 0.88 ± 0.12 mg CE/g extract. The aqueous leaf extract showed the highest amount of flavanol compounds whereas the hexane bud extract gave the lowest result.
In our research, methanol proved to be the most effective solvent for flavanol extraction. Lee et al., who examined the anti-inflammatory activity and phytochemical content in pomegranate peel, reported the total amount of flavanols as 257.0 ± 19.6 μg CE/mg [59]. The difference may be due to the different plant tissues used. As far as we know, no study has reported the total flavanol content in pomegranate leaves, buds, and flowers so far.
Total anthocyanin content in different pomegranate parts. We defined the total anthocyanin content in P. granatum leaf, bud, and flower extracts using the pH differential method. The flower extract in acidified methanol demonstrated the highest content, i.e., 7.88 ± 0.18 mg Cy3G/kg extract (cyanidin-3-glucoside equivalent). The total anthocyanin content varied from 0.03 ± 0.00 to 7.88 ± 0.18 mg Cy3G/ kg extract. The anthocyanin content in the flower extract was significantly higher than those in the leaf and the bud extracts (Table 6).
Our results were in agreement with those reported by Elfalleh et al. who revealed that pomegranate flowers had more anthocyanin than leaves [37]. In the same study, the acidified methanol was found to be more effective in extracting anthocyanin than methanol, water, ethanol, acidified ethanol, acidified water, and hexane. Similar results were also reported by Castañeda-Ovando et al. [58].
Total tannin content in different pomegranate parts. To identify the total tannin content in pomegranate leaf, bud, and flower extracts, we appealed to a regression equation of calibration curve (y = 0.0075x + 0.0179) and expressed the results as mg ECE/g extract (epicatechin equivalent). The values varied between 2.99 ± 0.08 and 16.07 ± 0.09 for different pomegranate tissues (Table 7). The highest amount of total tannin compounds was found in the methanol flower extracts.
Mekni et al. examined the phytochemical contents and antioxidant properties of leaves, flowers, and bark of four different pomegranate cultivars [57]. In their study, the total tannin content in Nabli and Gapsi flowers was higher than in other samples. On the other hand, Trabelsi et al. showed that the aqueous extract of pomegranate leaves contained more tannin than chloroform, ethyl acetate, ethanol, and acetone/water extracts [51]. In our research, methanol proved to be the best extraction solvent for tannin compounds. These results were in agreement with other studies, where methanol extracts of different plants were reported to contain more tannins [61, 62].
High-performance liquid chromatography (HPLC) of individual polyphenol compounds in different pomegranate parts. The identification and quantification of polyphenol compounds in pomegranate leaves, buds, and flowers were conducted using a HPLC analysis. Figure 1 illustrates the chromatograms of the solutions of all 18 standard phenolic compounds acquired at 250, 280, 320, and 360 nm.
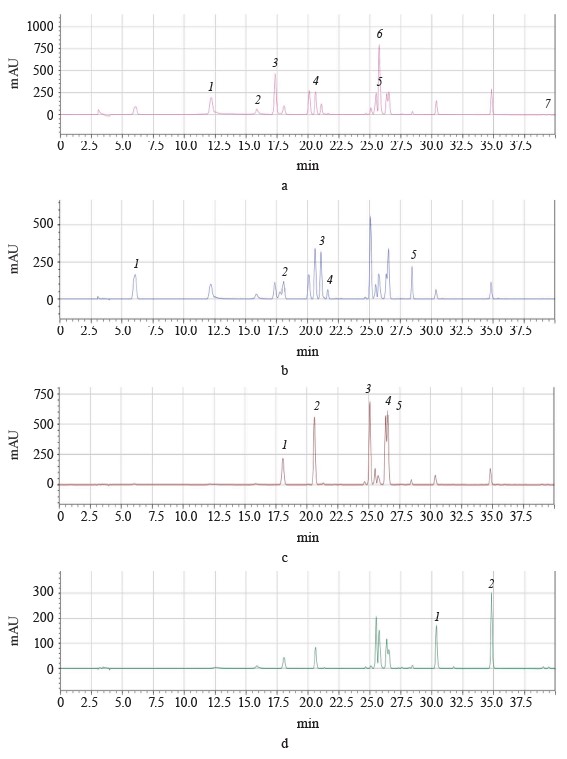
The HPLC analysis detected some major phenolic compounds, e.g., punicalagin in buds and flowers, as well as gallic acid in leaves. Gallic acid and ellagic acid were determined in all the methanol extracts of pomegranate leaves, buds, and flowers. However, punicalagin and protocatechuic acid were detected only in the methanol extracts of buds and flowers while punicalagin was more abundant than protocatechuic acid (Table 8).
We also tested the methanol extracts for snapic acid, trans-ferulic acid, caffeic acid, chlorogenic acid, myricetin, quercetin, naringin, p-coumaric acid, (-)-epicatechin, (+)-catechin, syringic acid, p-OH benzoic acid, rutin, and vanillic acid but detected none of them. In general, our results, except those obtained for the bud extracts, coincided with those reported by other authors [33, 51, 63, 64]. We found some studies that used HPLC to identify phenolic compounds in various buds but we found no article that reported pomegranate buds [65, 66].
2,2-diphenyl-1-picrylhydrazyl (DPPH) free radical scavenging activity in different pomegranate parts. We used the DPPH method to identify the free radical scavenging activity in different solvent extracts of pomegranate leaves, buds, and flowers (Figs. 2–4). As a rule, 2,2-diphenyl-1-picrylhydrazyl is employed as a substrate that reduces oxidative stress and hinders cellular ageing [67]. It helps evaluate the antioxidative activity of antioxidant substances. The assay relies on the reduction of methanolic or ethanolic DPPH solution in the presence of a hydrogen atom from the antioxidant. The reaction forms DPPH-H, a non-radical form of diphenylpicrylhydrazine [68]. In our research, all the extracts were able to reduce the stable radical DPPH to its yellow-stained non-radical form. The inhibition values of leaves, buds, and flowers ranged from 11.46 ± 1.13 to 85.68 ± 0.16%, from 17.74 ± 0.97 to 85.98 ± 0.16%, and from 9.60 ± 0.89 to 87.68 ± 0.89%, respectively.
While the acidified methanol extract of P. granatum flowers had the highest radical scavenging activity of 87.68 ± 0.89% at 125.0 μg/mL, the hexane extract of P. granatum flowers showed the lowest radical scavenging activity. All the extracts but the hexane one showed higher antioxidant activity than the synthetic antioxidant compounds used as standards, namely butylated hydroxyanisole (BHA), butylated hydroxytoluene (BHT), and α-tocopherol (α-TOC). At 12.5 μg/mL, which was the lowest concentration, the antioxidant activity of the acidified methanol bud extract was 1.8 and 4.7 times as high as that of butylated hydroxyanisole and the hexane bud extract. At 12.5 μg/mL, the pomegranate flower extract in acidified methanol showed approximately 8.8-fold higher activity than the hexane extract. All the extracts, excluding the hexane ones, exhibited a greater radical scavenging ability than the standard antioxidant compounds, even at the lowest concentration. No statistically significant differences were observed. We found several articles that featured the DPPH• free radical scavenging activities of P. granatum leaves, buds, and flowers [23, 29, 69].
The DPPH radical scavenging activity of pomegranate leaves ranged from 16.90 ± 0.46% at 20 μg/mL to 79.13 ± 0.37% at 120 μg/mL, probably, due to the diverse concentrations of methanol extract [23]. Yu et al. reported that the DPPH radical scavenging activity of hydro-methanolic extracts of pomegranate leaves was 4–200 times higher than the leaf extracts of Salvia officinalis L., Rosmarinus officinalis L., Olea europaea L., Ruta graveolens L., Mentha piperita L., and Petroselinum crispum Mill. [69]. In the study of Abdolahi et al., the radical scavenging activity in the pomegranate flower ethanol extract was 91.04% at 100 μg/mL [29]. This variation in the DPPH scavenging activity could be explained by the difference in plant varieties, growing environmental, and extraction conditions.
Ferrous ion chelating activity in different pomegranate parts. We used the assay described by Carter to determine the chelating activity of ferrous ions in different pomegranate extracts [48]. Metal chelating is frequently excogitated as the most accepted and widespread antioxidant method [70]. Ferrozine quantitatively forms complexes with Fe2+. In the presence of chelating agents, the complex formation is disrupted, and the purple staining of the complex decreases. By measuring the color reduction, researchers are able to estimate the chelating activity of the co-existing chelator [71]. In this method, both pomegranate extracts and ethylenediaminetetraacetic acid (EDTA) as chelating agent hindered the formation of ferrous complex with the reagent ferrozine, suggesting that pomegranate extracts possessed chelating activity and could capture the ferrous ion before ferrozine.
The absorbance of Fe2+-ferrozine complex went down in a dose-dependent manner in leaf, bud, and flower extracts as 12.5, 25.0, 37.5, 62.5, and 125.0 μg/mL, respectively. Butylated hydroxyanisole, butylated hydroxytoluene, and α-tocopherol showed less metal chelating activity than the pomegranate extracts and ethylenediaminetetraacetic acid. Figure 5 illustrates the metal chelating activity in the P. granatum leaves, butylated hydroxyanisole, butylated hydroxytoluene, α-tocopherol, and ethylenediaminetetraacetic acid at different concentrations.
The metal chelating activity of pomegranate leaf extracts varied from 4.71 ± 0.30 to 76.52 ± 0.89%. Statistically, the aqueous extracts had the highest chelating activity, followed by acidified water, methanol, acidified methanol, ethanol, acidified ethanol, and hexane extracts. The hexane leaf extracts displayed the lowest chelating activity. Figure 6 shows the metal chelating activity in P. granatum bud extracts, standard antioxidants, and ethylenediaminetetraacetic acid at different concentration.
The metal chelating activity of P. granatum bud extracts ranged from 0.14 ± 0.10 to 74.15 ± 1.01%. The aqueous bud extract exhibited the greatest metal chelating activity compared to other bud extracts. At 125.0 μg/mL, all the pomegranate flower extracts were highly capable of metal chelating, irrespectively of the solvent applied (Fig. 7).
The acidified methanol flower extracts showed the maximal metal chelating activity, compared to other solvents. The metal chelating activity of P. granatum flower extracts varied from 1.79 ± 0.28 to 70.90 ± 0.15%. Our metal chelation assay data were similar to other reports. For instance, Rummun et al. assessed the metal chelating properties of pomegranate leaves, peel, stems, seeds, and flowers to report that flowers exhibited higher activity than leaves [72]. We found no published reports describing metal chelating activity for P. granatum buds.
Reducing power ability in different pomegranate parts. We used the reducing power method to evaluate the reduction potential of different pomegranate extracts because this method comprises the reduction of ferricyanide ion to ferrocyanide ion by electron donation from phenolic compounds. Therefore, the reducing capability of a compound can function as an important indicator of its potential antioxidant activity. All extracts of pomegranate leaves, buds, and flowers clearly exhibit a dosedependent reducing power. Figures 8, 9, and 10 depict the reductive effects of different pomegranate extracts compared to butylated hydroxyanisole, butylated hydroxyxytoluene, and α-tocopherol. Similar to metal chelating activity, the reducing power of P. granatum leaf, bud, and flower extracts increased together with concentration.
The aqueous extracts of leaves demonstrated a higher reducing power compared to other solvents and synthetic antioxidant compounds. It was 2.06 ± 0.07 while that of butylated hydroxytoluene was 1.02 ± 0.02 at 44.11 μg/mL. The range of reducing power in P. granatum bud extracts varied from 0.11 ± 0.00 to 2.17 ± 0.03 at 5.88 and 44.11 μg/mL, respectively. In general, P. granatum bud extracts exhibited a higher reducing power than the antioxidant compounds like butylated hydroxytoluene and alpha-tocopherol. The highest reducing power value belonged to the acidified water extract, followed by the acidified methanol extract. The reducing power in all the P. granatum flower extracts but the hexane one was higher than that of butylated hydroxytoluene and alphatocopherol at 29.41 and 44.11 μg/mL (Fig. 10). The reducing power value ranged from 0.11 ± 0.02 at 5.88 μg/mL to 2.13 ± 0.04 at 44.11 μg/mL with the highest reducing power measured in the acidified methanol extracts.
Consequently, bud and flower extracts exhibited higher activities than leaf extracts. In addition, no significant difference was observed between the acidified water, water, methanol, and acidified methanol extracts of pomegranate leaves, buds and flowers. Our results were similar to those reported by other research teams [29, 39, 73]. For instance, Zhang et al. wrote that the reducing power of different parts of pomegranate flowers increased steadily following the increase in the extract concentration [73].
Total antioxidant activity in different pomegranate parts. In this research we used the CUPRAC method, i.e., cupric reducing antioxidant capacity. It is based on the ability of antioxidants to reduce the cupric neocuproine complex Cu (II)-neocuproine in the presence of ammonium acetate to the cuprous form Cu (I)-neocuproine chelate, which shows the maximal light absorption at 450 nm [50]. The leaf extracts gave antioxidant activity that ranged from 517.27 ± 1.40 to 3509.42 ± 0.40 μmol TRE/g extract (Trolox equivalent). In various bud extracts, this variable ranged from 385.60 ± 0.42 to 3323.09 ± 1.78 μmol TRE/g extract. In different flower extracts, it was between 350.66 ± 3.84 and 3282.58 ± 1.31 μmol TRE/g extract (Table 9).
Statistically, we determined that the total antioxidant activity of pomegranate buds exceeded that of the leaves and flowers. In our research, water proved to be the best extraction solvent in terms of the cupric reducing antioxidant capacity of pomegranate. Our results were similar to those obtained by some other researchers. For example, Ghazzawi et al. examined the effects of different solvents on the antioxidant capacity and content in nine seasonal fruits, including pomegranates [74]. In their study, water appeared to be the best extraction solvent for the antioxidant capacity of bananas, figs, white grapes, and jujube fruits. Sanda et al. also reported that the aqueous extract of D. pontica leaves exhibited the highest cupric reducing power capacity [75]. Alsataf et al. explored the antioxidant, anti-diabetic, and antimicrobial activities of pomegranate peel, mesocarp, and seed, as well as pomegranate juices [76]. In their research, the highest cupric reducing capacity belonged to pomegranate peel (954.4 μmol trolox/g). The researchers also reported that pomegranate tissues had a significant effect on anti-α-glucosidase activity and exhibited different antimicrobial activities against pathogens. Our values exceeded those obtained by Alsataf et al. [76]. This difference could be explained by the different pomegranate tissues involved. Uysal et al. performed the CUPRAC test on nine different fruit tree leaves [77]. The list included avocado, walnut, mulberry, fig, carob, lemon, pomegranate, grape, and loquat. In their study, the pomegranate leaf extracts had the highest CUPRAC absorbance values at the 0.1 mg/mL concentration. As far as we know, no study reported cupric reducing power activity of pomegranate buds and flowers so far.
ВЫВОДЫ
In this research, which featured pomegranate leaves, buds, and flowers, the phenolic content and antioxidant activity of plant extracts depended on the plant tissue and the extraction solvent involved. Punica granatum L. buds demonstrated the greatest polyphenol content, as well as antioxidant activity. The flower extracts were rich in tannin and anthocyanin. P. granatum leaves demonstrated higher flavanol content than other tissues.
The P. granatum bud extract showed higher antioxidant activity than leaves and flowers. Pomegranate buds, leaves, and flowers possessed beneficial polyphenol and antioxidant profiles, which made them potential raw material sources for pharmaceutical preparations, functional foods, nutraceuticals, and cosmetics.
Вклад авторов
The authors were equally involved in writing the manuscript and are equally responsible for any potential cases of plagiarism.
КОНФЛИКТ ИНТЕРЕСОВ
The authors declared no conflict of interests regarding the publication of this article.
ФИНАНСИРОВАНИЕ
The research was supported by the İnönü University Research Fund (TDK-2020-2100).СПИСОК ЛИТЕРАТУРЫ
- Das K, Roychoudhury A. Reactive oxygen species (ROS) and response of antioxidants as ROS-scavengers during environmental stress in plants. Frontiers in Environmental Science. 2014;2:53. https://doi.org/10.3389/fenvs.2014.00053
- Demirci-Çekiç S, Özkan G, Avan AN, Uzunboy S, Çapanoğlu E, Apak R. Biomarkers of oxidative stress and antioxidant defense. Journal of Pharmaceutical and Biomedical Analysis. 2022;209:114477. https://doi.org/10.1016/j.jpba.2021.114477
- Juan CA, Pérez de la Lastra JM, Plou FJ, Pérez-Lebeña E. The chemistry of reactive oxygen species (ROS) revisited: Outlining their role in biological macromolecules (DNA, lipids and proteins) and induced pathologies. International Journal of Molecular Sciences 2021;22(9):4642. https://doi.org/10.3390/ijms22094642
- Redza-Dutordoir M, Averill-Bates DA. Activation of apoptosis signalling pathways by reactive oxygen species. Biochimica et Biophysica Acta (BBA) – Molecular Cell Research. 2016;1863(12):2977–2992. https://doi.org/10.1016/j.bbamcr.2016.09.012
- Birben E, Sahiner UM, Sackesen C, Erzurum S, Kalayci O. Oxidative stress and antioxidant defense. World Allergy Organization Journal. 2012;5(1):9–19. https://doi.org/10.1097/WOX.0b013e3182439613
- Faskhutdinova ER, Sukhikh AS, Le VM, Minina VI, Khelef MEA, Loseva AI. Effects of bioactive substances isolated from Siberian medicinal plants on the lifespan of Caenorhabditis elegans. Foods and Raw Materials. 2022;10(2):340–352. https://doi.org/10.21603/2308-4057-2022-2-544
- Pizzino G, Irrera N, Cucinotta M, Pallio G, Mannino F. Arcoraci V, et al. Oxidative stress: Harms and benefits for human health. Oxidative Medicine and Cellular Longevity. 2017;2017:8416763. https://doi.org/10.1155/2017/8416763
- Sharifi-Rad M, Anil Kumar NV, Zucca P, Varoni EM, Dini L, Panzarini E, et al. Lifestyle, Oxidative stress, and antioxidants: Back and forth in the pathophysiology of chronic diseases. Frontiers in Physiology. 2020;11:694. https://doi.org/10.3389/fphys.2020.00694
- Xu X, Liu A, Hu S, Ares I, Martínez-Larrañaga M-R, Wang X, et al. Synthetic phenolic antioxidants: Metabolism, hazards and mechanism of action. Food Chemistry. 2021;353:129488. https://doi.org/10.1016/j.foodchem.2021.129488
- Wang W, Xiong P, Zhang H, Zhu Q, Liao C, Jiang G. Analysis, occurrence, toxicity and environmental health risks of synthetic phenolic antioxidants: A review. Environmental Research. 2021;201:111531. https://doi.org/10.1016/j.envres.2021.111531
- Lourenço SC, Moldão-Martins M, Alves VD. Antioxidants of natural plant origins: From sources to food industry applications. Molecules. 2019;24(22):4132. https://doi.org/10.3390/molecules24224132
- Kandylis P, Kokkinomagoulos E. Food applications and potential health benefits of pomegranate and its derivatives. Foods. 2020;9(2):122. https://doi.org/10.3390/foods9020122
- Dandachi F, Hamadeh B, Youssef H, Chahine H, Chalak L. Diversity assessment of the Lebanese germplasm of pomegranate (Punica granatum L.) by morphological and chemical traits. Annals of Agricultural Sciences. 2017;62(1):89–98. https://doi.org/10.1016/j.aoas.2017.05.004
- Karimi M, Sadeghi R, Kokini J. Pomegranate as a promising opportunity in medicine and nanotechnology. Trends in Food Science and Technology. 2017;69:59–73. https://doi.org/10.1016/j.tifs.2017.08.019
- Middha SK, Usha P, Pande V. HPLC evaluation of phenolic profile, nutritive content, and antioxidant capacity of extracts obtained from Punica granatum fruit peel. Advences in Pharmacological and Pharmaceutical Sciences. 2013;2013:296236. https://doi.org/10.1155/2013/296236
- Derakhshan Z, Ferrante M, Tadi M, Ansari F, Heydari A. Hosseini MS, et al. Antioxidant activity and total phenolic content of ethanolic extract of pomegranate peel, juice and seeds. Food and Chemical Toxicology. 2018;114:108–111. https://doi.org/10.1016/j.fct.2018.02.023
- Ricci D, Giamperi L, Bucchini A, Fraternale D. Antioxidant activity of Punica granatum fruits. Fitoterapia. 2006;77(4):310–312. https://doi.org/10.1016/j.fitote.2006.01.008
- Küçükbay FZ, Tekin Z. Evaluation of phytochemical contents and antioxidant activity of pomegranate flower. Journal of the Turkish Chemical Society Section A: Chemistry. 2020;7(1):37–42. https://doi.org/10.18596/jotcsa.628615
- Hafizov SG, Musina ON, Hafizov GK. Extracting hydrophilic components from pomegranate peel and pulp. Food Processing: Techniques and Technology. 2023;53(1):168–182. (In Russ.). https://doi.org/10.21603/2074-9414-2023-1-2425
- Cheurfa M, Achouche M, Azouzi A, Abdalbasit MA. Antioxidant and anti-diabetic activity of pomegranate (Punica granatum L.) leaves extracts. Foods and Raw Materials. 2020;8(2):329–336. https://doi.org/10.21603/2308-4057-2020-2-329-336
- Ge S, Duo L, Wang J, Zhula G, Yang J, Li Z, et al. A unique understanding of traditionally medicine of pomegranate, Punica granatum L. and its current research status. Journal of Ethnopharmacology. 2021;271:113877. https://doi.org/10.1016/j.jep.2021.113877
- Balamurugan C, Karuppasamy R, Sivaraj C, Saraswathi K, Arumugam P. Cytotoxic activity of leaves extract of pomegranate (Punica granatum L.). Journal of Pharmacognosy and Phytochemistry. 2021;10(1):1982–1985. https://doi.org/10.22271/phyto.2021.v10.i1ab.13640
- Balamurugan C, Karuppasamy R, Sivaraj C, Saraswathi K, Arumugam P. Punica granatum L. (Pomegranate) leaves extract: The study of antioxidant and antibacterial activity. Journal of Pharmacognosy and Phytochemistry. 2020;9(6):397–402. https://doi.org/10.22271/phyto.2020.v9.i6f.12915
- Bekir J, Mars M, Souchard JP, Bouajila J. Assessment of antioxidant, anti-inflammatory, anti-cholinesterase and cytotoxic activities of pomegranate (Punica granatum) leaves. Food and Chemical Toxicology. 2013;55:470–475. https://doi.org/10.1016/j.fct.2013.01.036
- Dassprakash MV, Arun R, Abraham SK, Premkumar K. In vitro and in vivo evaluation of antioxidant and antigenotoxic potential of Punica granatum leaf extract. Pharmaceutical Biology. 2012;50(12):1523–1530. https://doi.org/10.3109/13880209.2012.689771
- Huang THW, Peng G, Kota BP, Li GQ, Yamahara J, Roufogalis BD, et al. Anti-diabetic action of Punica granatum flower extract: Activation of PPAR-γ and identification of an active component. Toxicology and Applied Pharmacology. 2005;207(2):160–169. https://doi.org/10.1016/j.taap.2004.12.009
- Xu J, Zhao Y, Aisa HA. Anti-inflammatory effect of pomegranate flower in lipopolysaccharide (LPS) - stimulated RAW264.7 macrophages. Pharmaceutical Biology. 2017;55(1):2095–2101. https://doi.org/10.1080/13880209.2017.1357737
- Sarker M, Das SC, Saha SK, Al Mahmud Z, Bachar SC. Analgesic and anti-inflammatory activities of flower extracts of Punica granatum Linn. (Punicaceae). Journal of Applied Pharmaceutical Science. 2012;02(04):133–136. https://doi.org/10.7324/JAPS.2012.2408
- Abdolahi N, Soltani A, Mirzaali A, Soltani S, Balakheyli H, Aghaei M. Antibacterial and antioxidant activities and phytochemical properties of Punica granatum flowers in Iran. Iranian Journal of Science and Technology, Transactions A: Science. 2018;42:1105–1110. https://doi.org/10.1007/s40995-017-0413-8
- Celik I, Temur A, Isik I. Hepatoprotective role and antioxidant capacity of pomegranate (Punica granatum) flowers infusion against trichloroacetic acid-exposed in rats. Food and Chemical Toxicology. 2009;47(1):145–149. https://doi.org/10.1016/j.fct.2008.10.020
- Ahangarpour A, Heidari R, Abdolahzadeh M, Oroojan AA. Antispasmodic effects of aqueous and hydroalcoholic Punica granatum flower extracts on the uterus of non-pregnant rats. Journal of Reproduction and Infertility. 2012;13(2):138–142.
- Barwal SB, Nirmal Sunil A, Dhasade VV, Patil MJ, Pal SC, Mandal Subhash C. Antihistaminic effect of various extracts of Punica granatum Linn. flowers buds. Journal of Young Pharmacists. 2009;1(4):332–335. https://doi.org/10.4103/0975-1483.59321
- Orgil O, Schwartz E, Baruch L, Matityahu I, Mahajna J, Amir R. The antioxidative and anti-proliferative potential of non-edible organs of the pomegranate fruit and tree. LWT – Food Science and Technology 2014;58(2):571–577. https://doi.org/10.1016/j.lwt.2014.03.030
- Montefusco A, Durante M, Migoni D, de Caroli M, Ilahy R, Pék Z, et al. Analysis of the phytochemical composition of pomegranate fruit juices, peels and kernels: A comparative study on four cultivars grown in southern Italy. Plants. 2021;10(11):2521. https://doi.org/10.3390/plants10112521
- Boussaa F, Zaouay F, Burlo-Carbonell F, Noguera-Artiaga L, Carbonell-Barrachina A, Melgarejo P, et al. Growing location affects physical properties, bioactive compounds, and antioxidant activity of pomegranate fruit (Punica granatum L. var. Gabsi). International Journal of Fruit Science. 2020;20:508–523. https://doi.org/10.1080/15538362.2020.1741058
- Melgarejo-Sánchez P, Núñez-Gómez D, Martínez-Nicolás JJ, Hernández F, Legua P, Melgarejo P. Pomegranate variety and pomegranate plant part, revelance from bioactive point of view: A review. Bioresources and Bioprocessing. 2021;8:2. https://doi.org/10.1186/s40643-020-00351-5
- Dai J, Mumper RJ. Plant phenolics: Extraction, analysis and their antioxidant and anticancer properties. Molecules. 2010;15(10):7313–7352. https://doi.org/10.3390/molecules15107313
- Wakeel A, Jan SA, Ullah I, Shinwari ZK, Xu M. Solvent polarity mediates phytochemical yield and antioxidant capacity of Isatis tinctoria. PeerJ. 2019;7:e7857. https://doi.org/10.7717/peerj.7857
- Elfalleh W, Hannachi H, Tlili N, Yahia Y, Nasri N, Ferchichi A. Total phenolic contents and antioxidant activities of pomegranate peel, seed, leaf and flower. Journal of Medicinal Plants Research. 2012;6:4724–4730. https://doi.org/10.5897/JMPR11.995
- Singleton VL, Orthofer R, Lamuela-Raventos RM. Analysis of total phenols and other oxidation substrates and antioxidants by means of Folin-Ciocalteu reagent. Methods in Enzymology. 1999;299:152–178. https://doi.org/10.1016/S0076-6879(99)99017-1
- Zhishen J, Mengcheng T, Jianming W. The determination of flavonoid contents in mulberry and their scavenging effects on superoxide radicals. Food Chemistry. 1999;64(4):555–559. https://doi.org/10.1016/S0308-8146(98)00102-2
- Kumaran A, Karunakaran RJ. In vitro antioxidant activities of methanol extracts of five Phyllanthus species from India. LWT – Food Science and Technology. 2007;40(2):344–352. https://doi.org/10.1016/j.lwt.2005.09.011
- McMurrough I, McDowell J. Chromatographic separation and automated analysis of flavanols. Analytical Biochemistry. 1978;91(1):92–100. https://doi.org/10.1016/0003-2697(78)90819-9
- Lee J, Durst RW, Wrolstad RE. Determination of total monomeric anthocyanin pigment content of fruit juices, beverages, natural colorants, and wines by the pH differential method: Collaborative study. Journal of AOAC International. 2005;88(5):1269–1278. https://doi.org/10.1093/jaoac/88.5.1269
- Broadhurst RB, Jones WT. Analysis of condensed tannins using acidified vanilin. Journal of the Science of Food and Agriculture. 1978;29(9):788–794. https://doi.org/10.1002/jsfa.2740290908
- Gupta C, Verma R. Visual estimation and spectrophotometric determination of tannin content and antioxidant activity of three common vegetable. International Journal Pharmaceutical Sciences and Research. 2011;2(1):175–182. https://doi.org/10.13040/IJPSR.0975-8232.2(1).175-82
- Blois MS. Antioxidant determinations by the use of a stable free radical. Nature. 1958;181(4617):1199–1200. https://doi.org/10.1038/1811199a0
- Carter P. Spectrophotometric determination of serum iron at the submicrogram level with a new reagent (ferrozine). Analytical Biocehmistry. 1971;40(2):450–458. https://doi.org/10.1016/0003-2697(71)90405-2
- Oyaizu M. Studies on product of browning reaction. Antioxidative activities of products of browning reaction prepared from glucosamine. The Japanese Society of Nutrition and Dietetics. 1986;44(6):307–315. https://doi.org/10.5264/eiyogakuzashi.44.307
- Apak R, Güçlü K, Ozyürek M, Karademir SE. Novel total antioxidant capacity index for polyphenols and vitamins C and E, using their cupric ion reducing capability in the presence of neocuproine: CUPRAC method. Journal of Agricultural and Food Chemistry. 2004;52(26):7970–7981. https://doi.org/10.1021/jf048741x
- Trabelsi A, El Kaibi MA, Abbassi A, Horchani A, Chekir-Ghedira L, Ghedira K. Phytochemical study and antibacterial and antibiotic modulation activity of Punica granatum (Pomegranate) leaves. Scientifica. 2020;2020:8271203. https://doi.org/10.1155/2020/8271203
- Kaur G, Jabbar Z, Athar M, Alam MS. Punica granatum (pomegranate) flower extract possesses potent antioxidant activity and abrogates Fe-NTA induced hepatotoxicity in mice. Food and Chemical Toxicology. 2006;44(7):984–993. https://doi.org/10.1016/j.fct.2005.12.001
- Abubakar AR, Haque M. Preparation of medicinal plants. Basic extraction and fractionation procedures for experimental purposes. Journal of Pharmacy and BioAllied Sciences. 2020;12(1):1–10. https://doi.org/10.4103/jpbs.JPBS_175_19
- Kaneria MJ, Bapodara MB, Chanda SV. Effect of extraction techniques and solvents on antioxidant activity of pomegranate (Punica granatum L.) leaf and stem. Food Analytical Methods. 2012;5:396–404. https://doi.org/10.1007/s12161-011-9257-6
- Zheng J, Yu X, Maninder M, Xu B. Total phenolic and antioxidants profiles of commonly consumed edible flowers in China. International Journal of Food Properties. 2018;21(1):1524–1540. https://doi.org/10.1080/10942912.2018.1494195
- Attanayake R, Rajapaksha R, Weerakkody P, Bandaranayake PCG. The effect of maturity status on biochemical composition, antioxidant acitivity, and antocyanin biosynthesis gene expression in a pomegranate (Punica granatum L.) cultivar with red flowers, yellow peel, and pinkish arils. Journal of Plant Growth Regulation. 2019;38:992–1006. https://doi.org/10.1007/s00344-018-09909-2
- Mekni M, Azez R, Tekaya M, Mechri B, Hammami M. Phenolic, non-phenolic compounds and antioxidant activity of pomegranate flower, leaf and bark extracts of four Tunisian cultivars. Journal of Medicinal Plants Research. 2013;7(17):1100–1107.
- Kopjar M, Orsolic M, Pilizota V. Anthocyanins, phenols, and antioxidant activity of sour cherry puree extracts and their stability during storage. International Journal of Food Properties. 2014;17(6):1393–1405. https://doi.org/10.1080/10942912.2012.714027
- Lee C-J, Chen L-G, Liang W-L, Wang C-C. Anti-inflammatory effects of Punica granatum Linne in vitro and in vivo. Food Chemistry. 2010;118(2):315–322. https://doi.org/10.1016/j.foodchem.2009.04.123
- Castañeda-Ovando A, Pacheco-Hernández ML, Páez-Hernández ME, Rodríguez JA, Galán-Vidal CA. Chemical studies of anthocyanins: A review. Food Chemistry. 2009;113(4):859–871. https://doi.org/10.1016/j.foodchem.2008.09.001
- Iloki-Assanga SB, Lewis-Luján LM, Lara-Espinoza CL, Gil-Salido AA, Fernandez-Angulo D, Rubio-Pino JL, et al. Solvent effect on phytochemical constituent profiles and antioxidant activities, using four different extraction formulations for analysis of Bucida buceras L. and Phoradendron californicum. BMC Research Notes. 2015;8:396. https://doi.org/10.1186/s13104-015-1388-1
- Medini F, Fellah H, Ksouri R, Abdelly C. Total phenolic, flavonoid and tannin contents and antioxidant and antimicrobial activities of organic extracts of shoots of the plant Limonium delicatulum. Journal of Taibah University for Science. 2014;8(3):216–224. https://doi.org/10.1016/j.jtusci.2014.01.003
- Zheng J, Meenu M, Xu B. A systematic investigation on free phenolics acids and flavonoids profiles of commonly consumed edible flowers in China. Journal of Pharmaceutical and Biomedical Analysis. 2019;172:268–277. https://doi.org/10.1016/j.jpba.2019.05.007
- Feng L, Yin Y, Fang Y, Yang X. Quantitative determination of punicalagin and related substances in different parts of pomegranate. Food Analytical Methods. 2017;10:3600–3606. https://doi.org/10.1007/s12161-017-0916-0
- Ghafoor K, Al Juhaimi F, Özcan MM, Uslu N, Babiker EE, Mohamed Ahmed IA. Bioactive properties and phenolic compounds in bud, sprout, and fruit of Capparis spp. plants. Journal of Food Processing and Preservation. 2020;44(3):e14357. https://doi.org/10.1111/jfpp.14357
- Donno D, Turrini F, Boggia R, Guido M, Gamba G, Mellano MG, et al. Vitis vinifera L. pruning waste for bud-preparations as source of phenolic compounds-traditional and innovative extraction techniques to produce new natural products. Plants. 2021;10(11):2233. https://doi.org/10.3390/plants10112233
- Gang Y, Eom T-Y, Marasinghe SD, Lee Y, Jo E, Oh C. Optimising the DPPH assay for cell-free marine microorganism supernatants. Marine Drugs. 2021;19(5):256. https://doi.org/10.3390/md19050256
- Baliyan S, Mukherjee R, Priyadarshini A, Vibhuti A, Gupta A, Pandey RP, et al. Determination of antioxidants by DPPH radical scavenging activity and quantitative phytochemical analysis of Ficus religiosa. Molecules. 2022;27(4):1326. https://doi.org/10.3390/molecules27041326
- Yu M, Gouvinhas I, Rocha J, Barros AIRNA. Phytochemical and antioxidant analysis of medicinal and food plants towards bioactive food and pharmaceutical resources. Scientific Reports. 2021;11:10041. https://doi.org/10.1038/s41598-021-89437-4
- Gulcin İ, Alwasel SH. Metal ions, metal chelators and metal chelating assay as antioxidant method. Processes. 2022;10(1):132. https://doi.org/10.3390/pr10010132
- Gulcin İ, Buyukokuroglu ME, Kufrevioglu OI. Metal chelating and hydrogen peroxide scavenging effects of melatonin. Journal of Pineal Research. 2003;34(4):278–281. https://doi.org/10.1034/j.1600-079x.2003.00042.x
- Rummun N, Somanah J, Ramsaha S, Bahorun T, Neergheen-Bhujun VS. Bioactivity of nonedible parts of Punica granatum L.: A potential source of functional ingredients. International Journal of Functional Ingredients. 2013;2013:602312. https://doi.org/10.1155/2013/602312
- Zhang L, Yang X, Zhang Y, Wang L, Zhang R. In vitro antioxidant properties of different parts of pomegranate flower. Food and Bioproducts Processing. 2011;89(3):234–240. https://doi.org/10.1016/j.fbp.2010.04.007
- Ghazzawi HA, Al-Sayyed HF, Al-Kurd RA, Mwalla MM, Arafat TA, AbdelQader SM. Effect of different extraction solvents on the antioxidant content and capacity of nine seasonal fruits. Clinical Nutrition Open Science. 2021;38:33–42. https://doi.org/10.1016/j.nutos.2021.06.003
- Sanda MA, Zengin G, Aktumsek A, Cakmak YS. Evaluation of antioxidant potential of two Daphne species (D. gnidioides and D. pontica) from Turkey. Emirates Journal of Food and Agriculture. 2015;27(6):488–494. https://doi.org/10.9755/ejfa.2015.04.030
- Alsataf S, Başyiğit B, Karaaslan M. Mutivariate analyses of the antioxidant, antidiabetic, antimicrobial activity of pomegranate tissues with respect to pomegranate juice. Waste and Biomass Valorization. 2021;12:5909–5921. https://doi.org/10.1007/s12649-021-01427-9
- Uysal S, Zengin G, Aktumsek A, Karatas S. Chemical and biological approaches of nine fruit tree leaves collected from the Mediterranean region of Turkey. Journal of Functional Foods. 2016;22:518–532. https://doi.org/10.1016/j.jff.2016.02.006