Аннотация
In addition to studying bioactive organic compounds in plants, it is increasingly important to determine the biological role of elements in plants growing in environmentally unfavorable areas. One of such regions in Russia is Kuzbass with its intensively developing chemical, metallurgical, and coal mining sectors. In this study, we assessed the plant materials of red clover (Trifolium pratense L.), alsike clover (Trifolium hybridum L.), and white clover (Trifolium repens L.) collected from their natural populations in Kuzbass.The qualitative and quantitative composition of heavy metals in the clover samples was determined voltammetrically. The contents of molybdenum and phosphorus were measured by the photocolorimetric method. Total nitrogen and protein were determined by the Kjeldahl method. Nickel, cobalt, and chromium were quantified by spectrophotometry.
We analyzed the plant materials of the clover samples for heavy metals and found that the content of lead was the least in red clover and the highest in alsike clover. Copper varied in a larger range and was minimal in red clover compared to that in alsike and white clover. Zinc was found at higher concentrations of in white and red clover compared to that in alsike clover. The levels of cadmium exceeded the maximum permissible concentrations in all the clover samples. We also revealed that the clover samples contained different amounts of various amino acids, including arginine, valine, lysine, glycine, aspartic acid, and alanine.
The plant materials of the clover species growing in Kuzbass can be used to improve the fertility of soil and nitrogen regime. However, the clover species should not be used in bulk feed for farm animals because of high concentrations of cadmium.
Ключевые слова
Clover, Trifolium pratense L., Trifolium hybridum L., Trifolium repens L., soil, microelements, amino acids, heavy metals, plantsВВЕДЕНИЕ
Recently, researchers have taken increasing interest in the biological role of metals. In particular, there have been numerous studies of heavy metals, a group of chemical elements with a relative atomic mass of over 40. Standards have been established for the contents of certain metals that are toxic and therefore dangerous for living organisms. The human body contains 81 natural metals out of 92, including 10 vital elements, namely Fe, I, Cu, Zn, Co, Cr, Mo, Ni, Se, and Mn. Permissible concentrations of metals are beneficial for plants, animals, and humans, while their increased concentrations have a negative impact. Numerous studies have shown that metal effects vary depending on their content in the environment and their requirement for microorganisms, plants, animals, and humans. However, rapid industrialization and the resulting global technogenic environmental pollution have led to anomalies of elements (particularly, heavy metals) [1–3].
It is important to determine the contents of heavy metals in environmental objects. Stripping voltammetry is commonly used to measure zinc, cadmium, lead, and copper. In this study, we aimed to determine concentrations of heavy metals in the clover species growing in various parts of Kuzbass (Russia).The toxic effects of heavy metals depend on the level of soil pollution, as well as on the properties of a specific metal [4, 5]. In nature, metal ions coexist and form various combinations with each other. Therefore, their toxic effects can both increase (synergistic effects) and decrease (antagonistic effects) [6]. For example, a mixture of zinc and copper is five times more toxic (synergistic effect) than the sum of their toxicity values obtained arithmetically [7]. A combination of zinc and nickel has the same synergistic effect, while zinc and cadmium produce an antagonistic effect, jointly causing unidirectional harm.
Synergism and antagonism can also be observed in multicomponent mixtures of metals. Therefore, the toxicological effect of environmental pollution with heavy metals depends on the mixture of metals, as well as their concentrations and mutual impact on biota. On the other hand, heavy metals and their compounds can have harmful effects on living organisms. In particular, they can accumulate in human tissues and cause a number of diseases [8, 9]. Toxic metals are metals that bring no benefit to biological processes (e.g. lead or copper). However, some elements which usually have a toxic effect on living organisms (e.g. zinc or cadmium) can also have a beneficial effect.
Carbon, hydrogen, oxygen, and nitrogen are organogenic elements. Altogether, they make up 95% of dry plant tissue, with 45, 42, 6.5, and 1.5% of carbon, oxygen, hydrogen, and nitrogen, respectively. The remaining 5% includes macroelements, such as phosphorus, sulfur, potassium, calcium, magnesium, iron, aluminum, silicon, sodium, and others. Metals with concentrations of 0.001% or less are called microelements. They are essential elements that perform important functions in plant life. Their deficiency or excess in plants can cause a number of diseases and even lead to death.
The makeup of elements in plant tissues and organs depends on changes in environmental factors.
Phosphorus is found in the earth’s crust at a concentration of 0.08%. It plays an important role in cell energy and is involved in the phosphorylation of cellular proteins using protein kinase enzymes [10]. This mechanism controls many metabolic processes, since phosphate redistributes electrical charges in a protein molecule, changing its structural and functional properties.
Phosphorus deficiency in plants causes the leaves to become small and narrow, as well as changes their color to bluish-green. Moreover, it decreases the absorption of molecular oxygen and changes the activity of respiratory enzymes. On the other hand, excessive phosphorus stops plant growth, delays the ripening of the crop, and inhibits the synthesis of proteins and free nucleotides. Phosphorus interferes with the absorption and transport of iron in plants.
Potassium, an important inorganic osmotic compound, helps regulate water balance in plants. It also maintains the water status of plants by opening and closing leaf stomata [11]. Potassium is involved in the optimization and transport of photosynthesis, as well as in the activation of photosynthetic enzymes, contributing to the production and transport of carbohydrates [12, 13]. In addition, potassium makes plants more resistant to biotic stress (diseases associated with microorganisms) and abiotic stress (drought, low temperatures, salinity). Thus, plants should be provided with a sufficient amount of potassium, since it takes part in many metabolic processes and regulates the growth of plants.
We found no published data on the accumulation of microelements in red clover (Trifolium pratense L.), alsike clover (Trifolium hybridum L.), and white clover (Trifolium repens L.) depending on the precursor, stage of growth and development, or environmental conditions. Microelements are known to activate metabolic processes. Yet, there is hardly any information on their composition, unlike macroelements [14].
Molybdenum deficiency slows down the synthesis of amides, amino acids, and proteins [15, 16]. Of all plants, clover has the greatest need for molybdenum and often suffers from molybdenum starvation in the fields [11, 17]. Nitrogenase is the key enzyme that catalyzes the fixation of molecular nitrogen in nitrogen-fixing microorganisms (aerobic and anaerobic bacteria, cyanobacteria) and root nodules of legumes. This enzyme catalyzes three coupled reactions: recovery of substrates (N2, C2H2, etc.), ATP hydrolysis, and ATP-dependent hydrogen evolution. Nitrogen is bound and recovered on the Mo-Fe protein. Molybdenum protonation is an important step for nitrogen binding, which results in the release of molecular hydrogen. In plants, molybdenum is found mainly in the form of the МоО42– anion. This element has the ability to change valency and participate in complex compounds. Legumes contain large amounts of molybdenum and are capable of N2-fixation. Experimental studies have shown that molybdenum fertilizers increase the nitrogen content in the leaf part of N-deficient plants with nodule bacteria on the roots. However, nitrates can suppress nitrogenase activity in the plants of the Fabaceae family. This may be due to the interception of electrons and ATP molecules by nitrate reductase contained in nodule bacteria. Enzymes compete for molybdenum in nitrogenfixer cells and nodule bacteria, especially in the budding phase, when their synthesis is most active [18].
Iron is one of the main elements found in the Earth’s crust. Its content in plants varies widely from 18 to 3580 mg/kg of dry weight. Iron is a universal cofactor for the processes of growth and development, photosynthesis, hormone synthesis, and respiration [14]. Iron deficiency causes leaves to turn yellow (chlorosis) and rapidly drop.
In Kuzbass, plants of the Trifolium genus are the most important perennial plants of the Fabaceae family in forage production. These plants are widely distributed in the region’s meadows and sparse forests [19]. Тheir fodder value is determined by their high protein content. In addition to being a low-cost source of plant protein, these plants can effectively increase soil fertility due to nitrogen absorbed from the atmosphere with the help of nitrogen-fixing bacteria in the nodules of the root system. Clover, one of such plants, is therefore used as a protein and vitamin additive in animal feed. For this, it is harvested in the early phases of the growing season and dried. According to literature, clover has a high content of digestible protein, namely 160–165 g per feed unit. Therefore, about 2 kg of clover grass is equivalent to 1 kg of oat grain. The chemical composition of plant reserve nutrients depends not only on the species characteristics, but also on the growth conditions [20, 21]. Proteins, fats, and carbohydrates, including cellulose, water, and microelements, determine the nutritional value of feed raw material, while bioactive substances provide it with additional properties [17, 22–26]. Noteworthily, enzymes, vitamins, growth substances, alkaloids, phenolic compounds, glycosides, tannins, and other essential bioactive substances play an important role in the processes of growth and development [27–30]. However, there are also toxic substances that can slow down the growth and development of plants and cause many pathological processes in them.
In recent years, Russian scientists have paid great attention to plant raw materials with a high feed value. We know that the plant’s biomass directly depends on the content of total nitrogen in the plant [31, 32]. Total nitrogen and protein play a major role in plant growth [33]. No other nutrient can affect food supplies as much as nitrogen, which is why it is called a “growth element” [34, 35]. First of all, it is an essential component of nitrogencontaining organic compounds and is actively involved in the vital metabolic processes during the plant’s growth and development. Nitrogen deficiency makes the plant look sickly, changes the color of its leaves, slows down its growth, weakens the intensity of flowering, shortens the period of growth and development, and reduces protein production in the plant. All this decreases the yield and may even cause the plant to die [36].
Nitrogen exchange reactions are determined not only by molybdenum and iron, but also by other metals, including nickel, cobalt, and chromium. These metals affect the ratio of nitrogen forms and the amino acid composition in plant raw materials [9].
Cobalt is found in small quantities in all soils, with larger quantities found in sedimentary rocks. This element accumulates in plants, ranging from 17 to 540 mg/kg ash. Cobalt can be found in different forms, e.g., in ionic form or as part of vitamin В12. Its biological role is to activate glycolytic enzymes (phosphoglucomutase and agrinase), which are involved in the hydrolysis of arginine. Both a deficiency and an excess of this metal can have a negative effect on plant development. Cobalt deficiency reduces the content of chlorophyll and ascorbic acid, while its excess inhibits biosynthesis and lowers the content of protein nitrogen in crops.
The lithosphere and soil are rich in nickel. This microelement promotes the production of vitamin P, increases the activity of redox enzymes, and stabilizes the structure of ribosomes. Nickel deficiency decreases photosynthetic activity in plants, while its excess can cause necrosis and chlorosis of the green parts of plants.
Chromium is found in various minerals and accumulates in the thin surface layer of soil. This metal is inaccessible to plants. Its content in dry plant mass does not usually exceed 0.02–0.2 mg/kg. Chromium has a dual effect on plant growth and development. On the one hand, this metal is part of many plant enzymes and therefore it affects the biosynthesis of flavonoids and promotes the accumulation of polysaccharides, tannins, carotenoids, cardiac glycosides, and vitamin K. On the other hand, chromium has phytotoxic properties which cause wilting of the aerial parts, damage the root system, and may lead to chlorosis and browning of young leaves. Chromium is also an antagonist of copper, manganese, and boron.
Zinc accumulates in the surface layers of soil and can also be found in some minerals. Its content in plants is 1.2–73.0 mg/kg of dry weight. Like cobalt, zinc ensures the functioning of some enzymes in the glucose oxidation process. Also, zinc plays an important role in the photosynthesis and promotes the production of tryptophan. Its deficiency may disturb phosphorus metabolism, retard the growth of internodes and leaves, as well as cause chlorosis, the development of rosettes, and the accumulation of reducing sugars.
Zinc is a unique metal due to its extensive biogenic properties. It is contained in more than 200 enzymes belonging to all six classes and therefore performs a variety of physiological functions. It is found in all 20 nucleotidyl transferases, as well as in reverse transcriptases. The presence of zinc in these compounds indicates a close relationship with the processes of tumor formation. In addition, zinc stabilizes the structure of DNA, RNA, and ribosomes, as well as plays an important role in gene translation and expression.
Elevated concentrations of zinc have a toxic effect on living organisms. Its excessive accumulation is closely related to anthropogenic activities, which is why high concentrations of zinc are found in industrial areas and agricultural soils over-treated with zinc-containing fertilizers. Many plant species can adapt to zinc excess in soils, but very high levels of this metal can still have a toxic effect. Chlorosis of young leaves is the most common symptom of zinc damage. Moreover, excessive levels of zinc decrease the rate of cell division in plant tissues, as well as impair cell elongation and tissue differentiation. Due to the antagonistic action of zinc towards other metals, its excess in plants reduces the absorption of copper and iron, causing symptoms of their deficiency. Zinc is also an antagonist of cadmium, selenium, and manganese.
Manganese is ubiquitous in rocks and soils. Its content in plants varies from 30 to 500 mg/kg of dry weight. Manganese plays an important biological role in plant growth and development. It is involved in the release of oxygen and carbon dioxide reduced during photosynthesis, regulates the sugar content in leaves, and participates in the processes of cell growth. Manganese deficiency has a negative effect on the plant, changing its morphology. In particular, it can cause spot chlorosis in the leaves (yellow spots) and tissue necrosis. High manganese contents cause a release of carbon dioxide from plants. Manganese does not interact with calcium or magnesium, but actively interacts with silicon. Manganese is high in plants that synthesize phenolic compounds, tannins, flavonoids, cardiac glycosides, ascorbic acid, carotenes, and vitamin K. Thus, the amount of manganese in clover can be used to estimate its yield and the content of bioactive substances in it. Also, manganese has a beneficial effect on the development and yield of clover seeds.
Copper is an immobile element found in the surface layer of soils. It is part of some proteins and enzymes that accelerate the oxidation of ascorbic acid and diphenols, as well as the hydroxylation of monophenols. Copper also participates in nitrogen-containing metabolism. Its deficiency contributes to stunted growth and flowering, as well as causes chlorosis, loss of turgor, and wilting of plants. Copper excess, on the other hand, affects the metabolism of organic acids and lengthens the period of plant growth and development. The average copper content in plants is 0.6–10.3 mg/kg of air-dry mass. Copper is actively involved in photosynthesis and respiration, as well as nitrogen recovery and fixation in plants. It participates in biochemical processes as part of some oxidase enzymes (e.g., cytochrome oxidase, ceruloplasmin, superoxide dismutase, urate oxidase) that carry out oxidation of substrates with molecular oxygen.
Recently, copper deficiency in soils or its antagonistic interaction with cobalt have been the main causes of concern. Some common signs of copper deficiency in plants include slow formation of reproductive organs and its subsequent cessation, the appearance of puny grains and empty-grained ears, as well as poor adaptation to unfavorable environmental conditions [37]. Wheat, barley, oats, beets, alfalfa, onions, and sunflowers are most sensitive to copper deficiency.
Although lead is found in almost all plants, scientists still lack a full understanding of its role in metabolic processes. According to literature, its low contents inhibit metabolism, while high concentrations have a toxic effect on plants by disrupting photosynthesis and slowing down growth and water absorption by the root system. External negative symptoms include the appearance of dark green leaves, as well as curled old leaves and stunted foliage. Plants exhibit differing resistance to excessive lead concentrations, with cereals being less resistant and legumes being more resistant. Lead has an antagonistic relationship with zinc and a synergic relationship with calcium. The lead content in plants reaches 0.1–10.0 mg/kg of dry weight.
Literature lacks reliable data on the value of cadmium for terrestrial plants. Its toxic effects include slow growth and photosynthesis, inactivated enzymes, disrupted water balance, inhibited reduction of NО2 to NO, as well as chlorosis [38]. In plant metabolism, cadmium is an antagonist of a number of nutrients (Zn, Cu, Mn, Ni, Se, Ca, Mg, and P). Cadmium is easily transported into plants from the soil and atmosphere. It is the most phytotoxic heavy metal that accumulates in plants (Cd > Cu > Zn > Pb).
Biotechnological methods are widely applied today to determine the nutritional value of plant materials [39, 40]. In studies of plants for animal feeds, great attention must be paid to the content of proteins, since they are functional units of a living cell that are absorbed in the form of amino acids after digestion (MR № 2.3.1.191504). Further, these amino acids get involved in plastic metabolism, ensuring the synthesis of their own proteins and other organic compounds [41].
In connection with the above, we carried out a comparative phytochemical study of red clover, alsike clover, and white clover growing in natural populations in Kuzbass. In particular, we performed qualitative and quantitative determination of macro- and microelements, heavy metals, free nitrogen, protein, and amino acids in the experimental plant speciesОБЪЕКТЫ И МЕТОДЫ ИССЛЕДОВАНИЯ
We studied above-ground parts of three types of clover, red clover (Trifolium pratense L.), white clover (Trifolium repens L.), and alsike clover (Trifolium hybri- dum L.) (Fig. 1). The materials were collected in the natural phytocenoses of Kuzbass (Russia) from the spring of 2023 throughout the flowering period in the summer of 2023. They were selected on the basis of physical factors (adult healthy plants) and visual characteristics (with no toxic effects of anthropogenic pathogens) by using standard methods [42].
The elemental composition of nickel, cobalt, and chromium was determined by spectrophotometry. This method works by evaporating the substance under analysis, photographing the emission spectrum of the elements excited in the arc discharge zone, and measuring the intensity of the spectral lines of the elements by visually comparing them with those for the reference powders and standard samples. The elements were determined by using atlases of spectral lines and standard spectra.
Cadmium and lead are classified as highly hazardous substances, while copper and zinc are moderately hazardous. Therefore, highly sensitive and accurate methods should be used to measure the contents of these elements. Among such methods is stripping voltammetry that can determine trace amounts. Another advantage of this method is that it can simultaneously determine several components (up to four or five). In addition, a modern polarograph can obtain a linear dependence of the current on concentration in the range of 10–8–10–2М.
Stripping voltammetry is an electrochemical method that includes the following stages:
1. Electrolytic accumulation of metal ions with a deposit or a metal film forming on the solid electrode coating of the amalgam or on the mercury film electrode. For this, it is important to maintain the rate of the electrode reaction by selecting a constant potential, as well as to constantly stir the solution to ensure a constant flow of the depolarizer from the solution to the electrode surface;
2. A short period of rest of the solution with accumulation potential. The flow moves to the electrode less intensively, causing a rapid drop to the value of the stationary diffusion current; and
3. Electrochemical dissolution of the substance from the electrode surface in the mode of linear potential sweep and strict stabilization of the current flowing between the indicator electrode and the auxiliary electrode.
The current of the dissolution of the concentrated electroactive substance is recorded as a peak. The peak’s potential characterizes the nature of the substance, its area indicates the quantity of the substance on the electrode, and its height relates to its concentration.
Stripping voltammetric methods can be anodic (reductive) or cathodic (oxidative).
The height of the peak depends on:
a) the amount of substance deposited on the electrode (which is a function of its concentration in the solution, accumulation potential, solution composition, temperature, and electrochemical properties of the system);
b) the conditions of the dissolution process (the area of the active electrode surface, the rate of polarization, and the rate of removal of products from the electrode surface).
Other factors may also influence the peak’s height. If the overall electrode process involves a chemical reaction, the peak is determined by the catalytic ability of this reaction, the nature of the reaction products, and the solubility of the resulting compounds.
Stripping voltammetry is a highly sensitive method that can determine nanogram quantities. It is applied to determine low concentrations up to 10–2 М, since larger amounts of the isolated sediment saturate the surface, causing a deviation from the direct proportional dependence of the current strength on the concentration. With low concentrations, the error does not exceed 3%.
In stripping voltammetry, just as in polarography, oxygen needs to be extracted from the solution under analysis. This is because at normal temperature and pressure, oxygen is relatively soluble in aqueous solutions and can interfere with the measurement.
Dissolved oxygen is electrolytically reduced, which increases the residual current whose value is poorly reproduced. The presence of dissolved oxygen can lead to other complications in electrochemical measurements. Therefore, in most cases, it is removed by passing through any inert gas that does not require purification. Other methods of oxygen removal (СО2 in acidic solutions, solid Na2S in alkaline solutions) have limited use. An inert gas must be passed over the solution throughout the entire analysis. It is important that inert gases should be free of oxygen. UV irradiation is also used in modern methods to remove dissolved oxygen. Thus, stripping voltammetry has high analytical potential and is employed to determine organic and inorganic compounds of various compositions.
In our experiment, Zn, Cd, Pb, and Cu were determined under optimal conditions in aqueous solutions against 0.5 M formic acid under UV irradiation to eliminate the interference of dissolved oxygen. During the analysis, interfering organic substances were destroyed in the electrochemical cell. We selected and tested the conditions for a joint determination of Zn, Cd, Pb, and Cu on a mercury film electrode in a solution of 0.5 M HCOOH with UV irradiation. The optimal conditions included an accumulation potential of 1.4 V and an accumulation time of 30 s for metal ion concentrations in the solution of 0.001–0.05 mg/L. Ion concentrations were determined by adding standard solutions. The air was aspirated through an aerosol filter form perchlorovinyl fiber at a rate of 20 L/min for 25 min.
Аmino acids, flavonoids, polyphenols, glycosides, and tannins were determined in the Laboratory for Biotesting of Natural Nutraceuticals at Kemerovo State University. The analysis was performed on a Prominence Shimadzu LC-20 high-performance liquid chromatography system with an SPD-M20A diode array detector and an RF-20 A/20Axs fluorimetric detector.
Total nitrogen and protein were measured by the Kjeldahl method according to General Monograph 1.2.3.0011.15. (OFC.1.5.3.0009.15).
Protein hydrolysates were analyzed by high-performance ion exchange chromatography using a special ninhydrin reagent for the demodulation of eluting amino acids. The samples of plant materials were crushed, weighed, and subjected to complete acid hydrolysis. The resulting hydrolysates were then analyzed.
To prepare the clover materials for the above analyses, they were placed in a thin layer on sheets of parchment paper, covered from dust with another sheet of paper, and left indoors at room temperature for 1–2 days to become air-dry (Fig. 2). Then, the samples were crushed into particles of under 1 cm and distributed on paper in the form of a square. The resulting square was then divided by horizontal and vertical lines into four equal parts, from which samples were taken with a spatula for the laboratory analyses. The weight of the samples depended on the number of analyses, ranging from 50 to 200 g. The samples were then mixed into average samples and placed into labelled glass jars with a ground stopper. In this state, the samples can be stored for a long time, with no change in moisture.
РЕЗУЛЬТАТЫ И ИХ ОБСУЖДЕНИЕ
The chemical compositions of the clover species growing in their natural populations in Kuzbass were analyzed for valuable feeding qualities(Fig. 3).
As for macroelements, the contents of phosphorus and potassium were almost identical between the clover samples under study (Table 1 and Fig. 4).
As seen from Table 1, the ratios of phosphorus to potassium did not differ significantly.
In particular, the phosphorus content was the highest (6.99 mg/kg) in alsike clover (Trifolium hybridum L.) and the lowest (6.48 mg/kg) in red clover (Trifolium prase L.). Potassium was noticeably higher (38.4 mg/kg) in white clover (Trifolium repens L.) and lower (36.6 mg/kg) in alike clover (Trifolium hybridum L.).
The ratio of molybdenum to iron in the clover samples under study is presented in Table 2, while the contents of these elements are shown in Fig. 5.
As can be seen, the content of molybdenum was the highest in red clover (T. pratense L.), slightly lower in white clover (T. repens L.), and the lowest in alike clover (T. hybridum L.). According to Fig. 5, iron had the highest content (168.51 mg/kg) in white clover and the lowest (135.04 mg/kg) in alsike clover.
One of the advantages of clover over perennial grasses is its higher yield due to nitrogen absorbed by symbiotic nodule bacteria from the atmospheric air. In addition, clover produces its own proteins due to biological nitrogen fixation without any energy costs or expensive nitrogen fertilizers. The ratio of total nitrogen to protein in the experimental clover species ranged from 5.87 to 6.43% (Table 3).
However, we found no direct relationship between the contents of total nitrogen and protein in the clover samples (Fig. 6). For example, white clover (T. repens L.) had the highest total nitrogen content of 3.802% and a protein content of 22.73%, while red clover (T. pratense L.) had a lower total nitrogen content of 3.715% and a higher protein content of 23.88%. Alsike clover (T. hybridum L.) showed the lowest total nitrogen content of 3.683% and the lowest protein content of 21.64%, compared to the other species under study.
Noteworthily, the clover genus of the Fabaceae family produces up to 80% of protein due to total nitrogen absorbed from the air by nodule bacteria of the root system. This is one of the main advantages of legumes over cereals.
We compared the contents of nickel, cobalt, and chromium as particularly toxic elements with the maximum permissible concentrations established in Sanitary Regulations 2.3.2.10733338-01 for black, green, and brick teas (Figs. 7–9).
As can be seen in Fig. 7, the sample of red clover contained nickel, cobalt, and chromium in permissible concentrations [10, 24, 28].
According to Fig. 8, the sample of white clover contained lower concentrations of these heavy metals than red clover.
As shown in Fig. 9, alsike clover (T. hybridum L.) had the lowest concentrations of nickel, cobalt, and chromium, compared to the other samples.
To sum up, all the clover samples complied with Sanitary Regulations 2.3.2.10733338-01. In particular, red clover (T. pratense L.) contained the maximum values of chromium, cobalt, and nickel; white clover (T. repens L.) showed an average value for nickel, while alsike clover (T. hybridum L.) showed the minimum values for all three metals. Cobalt had almost the same content in all the species, whereas nickel values varied from 3.5 mg/kg in red clover (T. pratense L.) to 3.1 mg/kg in alsike clover (T. hybridum L.). Thus, the plant materials of the clover species under study do not accumulate chromium, cobalt, and nickel in elevated concentrations. Therefore, these elements have no effect on the plants’ growth energy, viability and stability, the synthesis of highmolecular proteins, or the formation of vegetative mass.
The experimental values of heavy metals in the clover species under study are presented in the form of voltammograms in Figs. 10–12.
The voltammograms (Figs. 10–12) indicate the presence of Zn, Cd, Pb, and Cu in almost all the samples of the experimental clover species. The metrological characteristics for the determination of these heavy metals are presented in Tables 4–6.
We analyzed the contents of Zn, Cd, Pb, and Cu as particularly toxic elements against the maximum permissible concentrations established in Sanitary Regulations 2.3.2.10733338-01 for black, green, and brick teas [34].
According to Table 4, the contents of lead, copper, and zinc in the red clover (T. pratense L.) sample were within the permissible levels. However, the cadmium value was over six times the maximum permissible concentration.
As can be seen in Table 5, the sample of white clover (T. repens L.) contained lead, copper, and zinc in permissible concentrations, while its content of cadmium was over three times above the maximum level.
As shown in Table 6, the contents of lead, copper, and zinc in the alsike clover (T. hybridum L.) sample were within the permissible levels. However, its cadmium value was six times as high as the maximum permissible concentration established in Sanitary Regulations 2.3.2.10733338-01.
To sum up, the contents of lead, zinc and, copper in all the experimental clover samples were within the permissible concentrations by Sanitary Regulations 2.3.2. 10733338-01. Cadmium exceeded the standard values in all the three clover species. Zinc was found in almost equal amounts in red clover (T. pratense L.) and alsike clover (T. hybridum L.), with the maximum value in white clover (T. repens L.). The lead content ranged from 3.1×10–7 mg/L for white clover (T. repens L.) to 5.6×10–7 mg/L for alsike clover (T. hybridum L.). Copper varied from 3.2×10–7 mg/L for alsike clover (T. hybridum L.) to 5.1×10–7 mg/L for red clover (T. prаtense L.).
Thus, the plant materials of the studied clover species do not accumulate lead, copper, and zinc in elevated concentrations. Therefore, these metals have no effect on the plants’ growth, viability, and stability, as well as the synthesis of high-molecular proteins or the formation of vegetative mass. The elevated cadmium content leads to a lower plant yield and a deteriorated quality of plant products. The accumulation of heavy metals in plant materials has industrial causes. Today, the environment is becoming more and more chemically “aggressive”. Cadmium enters the soil in a mobile form and therefore rapidly migrates to plants, producing a negative effect on the environment.
The nutritional value of proteins is determined by the quality and quantity of individual amino acids that proteins are made of. Literature lacks data on the composition of amino acids in the clover species growing in Kuzbass. Therefore, at the final stage of our study, we determined the composition of amino acids in the plant materials of the experimental clover species (Fig. 13 and Table 7).
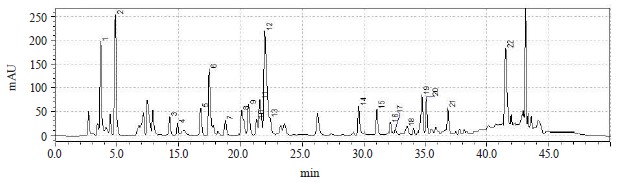
Red clover is one of the most common plants found in the European part of the former USSR, as well as throughout Western and Eastern Siberia, in a wide variety of environmental conditions, except for marshlands. This is a valuable forage plant with good feeding qualities, which is especially important in places with wet and slightly acidic soils capable of withstanding short-term flooding. Therefore, our next stage was to experimentally determine the content of amino acids in red clover grass.
We found that the experimental samples of the clover species had significant differences in the amino acid composition. According to Table 7, the alsike clover sample had maximum concentrations of all amino acids under study, with the exception of glutamic acid, which was the highest in the white clover sample. Thus, the clover species growing in Kuzbass can provide livestock with natural feed of high quality due to a wide variety of amino acids in the plants.
Chromatographic analysis was then performed to determine the contents of bioactive substances in the extract of red clover (Fig. 14 and Table 8).
Six substances were found in the red clover extracts, which were chromatographically classified as flavonoids. The following isoflavonoids were identified (in descending order): daidzein, biochanin A, quercetin, genistein, rutin, and apigenin. Their contents varied from 0.4300 mg/g for apigenin to 6.3400 mg/g for daidzein. All of these flavonoids can be successfully used as bioactive substances for therapeutic and preventative purposes.
ВЫВОДЫ
We studied the plant materials of red clover (Trifolium pratense L.), white clover (Trifolium repens L.), and alsike clover (Trifolium hybridum L.) growing in Kuzbass. Our experiments showed no significant differences in their nitrogen content.
The contents of phosphorus and potassium did not differ significantly among the clover species, ranging from 6.48 to 6.99 mg/kg and from 36.6 to 38.4 mg/kg, respectively. The content of cadmium significantly exceeded the maximum permissible concentrations in all the samples, while the contents of zinc and copper were experimentally determined to be minimal. The lead concentration was slightly higher than the standard values in red clover (T. pratense L.) and white clover (T. repens L.), reaching 10.7 and 11.0 mg/kg, respectively, however, a significant excess of lead (17.5 mg/kg) was detected in alsike clover (T. hybridum L.).
The protein content in the clover samples ranged from 21.64 to 23.88%. Such minor differences are due to a high content of total nitrogen synthesized by nodule bacteria in the roots of leguminous plants, on the one hand, and a high content of nitrogen accumulated during the decomposition of root residues during the growing season, on the other hand. All the clover samples had significant amounts of essential amino acids, such as tryptophan, lysine, leucine, isoleucine, methionine, phenylalanine, and valine. This variety of amino acids, and therefore complete protein, makes the clover a valuable forage plant. The total amount of amino acids was 245.1 mg/kg in red clover (T. pratense L.), 276.0 mg/kg in white clover (T. repens L.), and as much as 296.9 mg/kg in alsike clover (T. hybridum L.).
Thus, the plant materials of red, white, and alsike clover growing in Kuzbass had significant amounts of mineral substances (phosphorus, potassium, molybdenum, and iron), total nitrogen, and protein with essential amino acids. Their contents of particularly toxic elements such as nickel, lead, cobalt, chromium, copper, and zinc did not exceed permissible concentrations. From this we can conclude that these plants can be reliably used to improve the soil’s fertility and nitrogen regime. However, the concentration of cadmium in the clover samples was found to be over six times as high as the maximum permissible concentration. This can be explained by the presence of cadmium in fuel and fertilizers, as well as its use in the processing of coal and industrial waste in Kuzbass. Therefore, we would not recommend using the plant materials of the clover species to produce bulk feed for farm animals until the industrial processes in the region become more environmentally friendly and safe.Вклад авторов
All the authors are equally responsible for the research results and the manuscript.КОНФЛИКТ ИНТЕРЕСОВ
The authors declare no conflict of interest.ФИНАНСИРОВАНИЕ
This study was part of the Russian Science Foundation (RSF) project “Fundamentals of obtaining bioactive substances from Siberian medicinal plants and creating phytogenic feed additives with immunomodulatory effects on their basis” (Agreement No. 23-16-00113 dated May 15, 2023).СПИСОК ЛИТЕРАТУРЫ
- Aslam M, Aslam A, Sheraz M, Ali B, Ulhassan Z, Najeeb U, et al. Lead toxicity in cereals: Mechanistic insight into toxicity, mode of action, and management. Frontiers in Plant Science. 2021;11. https://doi.org/10.3389/fpls.2020.587785
- Chen G, Li J, Han H, Du R, Wang X. Physiological and molecular mechanisms of plant responses to copper stress. International Journal of Molecular Sciences. 2022;23(21). https://doi.org/10.3390/ijms232112950
- Asyakina LK, Dyshlyuk LS, Prosekov AYu. Reclamation of post-technological landscapes: International experience. Food Processing: Techniques and Technology. 2021;51(4):805–818. (In Russ.). https://doi.org/10.21603/2074-9414-2021-4-805-818
- Budantsev AL. Plant resources of Russia: Wild flowering plants, their composition and biological activity. Vol. 2. The families Actinidiaceae – Malvaceae, Euphorbiaceae – Haloragaceae. St. Petersburg, Moscow: KMK; 2009. 513 p. (In Russ.).
- Nizamutdinov ТI, Suleymanov AR, Morgun EN, Dinkelaker NV, Abakumov EV. Ecotoxicological analysis of fallow soils at the Yamal experimental agricultural station. Food Processing: Techniques and Technology. 2022;52(2):350–360. https://doi.org/10.21603/2074-9414-2022-2-2369
- Titov AF, Talanova VV, Kaznina NM, Laidinen GF. Plant resistance to heavy metals. Petrozavodsk; 2007. 170 p. (In Russ.).
- Li X, Wang Z, Bai M, Chen Z, Gu G, Li X, et al. Effects of polystyrene microplastics on copper toxicity to the protozoan Euglena gracilis: Emphasis on different evaluation methods, photosynthesis, and metal accumulation. Environmental Science and Pollution Research. 2022;29:23461–23473. https://doi.org/10.1007/s11356-021-17545-9
- Pelikhovich YuV, Begday IV, Kharin KV, Tsesar TA. Heavy metal accumulation in medicinal plants and risk assessment. Science. Innovations. Technologies. 2020;(4):171–183. (In Russ.). https://doi.org/10.37493/2308-4758.2020.4.13
- Goncharuk EA, Zagoskina NV. Heavy metals, their phytotoxicity, and the role of phenolic antioxidants in plant stress responses with focus on cadmium: Review. Molecules. 2023;28(9). https://doi.org/10.3390/molecules28093921
- Capuozzo M, Santorsola M, Bocchetti M, Perri F, Cascella M, Granata V, et al. p53: From fundamental biology to clinical applications in cancer. Biology. 2022;11(9). https://doi.org/10.3390/biology11091325
- Dʹyakova NA. The content of heavy metals and arsenic in the medicinal plants of the Voronezh region. Russia patent RU 2022620084. 2022. https://elibrary.ru/AAJXDT
- Ho L-H, Rode R, Siegel M, Reinhardt F, Neuhaus HE, Yvin J-C, et al. Potassium application boosts photosynthesis and sorbitol biosynthesis and accelerates cold acclimation of common plantain (Plantago major L.). Plants. 2020;9(10). https://doi.org/10.3390/plants9101259
- Li Y, Yin M, Li L, Zheng J, Yuan X, Wen Y. Optimized potassium application rate increases foxtail millet grain yield by improving photosynthetic carbohydrate metabolism. Frontiers in Plant Science. 2022;13. https://doi.org/10.3389/fpls.2022.1044065
- Chen J, Zhang N-N, Pan Q, Lin X-Y, Shangguan Z, Zhang J-H, et al. Hydrogen sulphide alleviates iron deficiency by promoting iron availability and plant hormone levels in Glycine max seedlings. BMC Plant Biology. 2020;20. https://doi.org/10.1186/s12870-020-02601-2
- Dyshlyuk LS, Osintseva MA. Kozlova OV, Fotina NV, Prosekov AYu. Antiradical and oxidative stress release properties of Trifolium pratense L. extract. Journal of Experimental Biology and Agricultural Sciences. 2022;10(4):852–860. https://doi.org/10.18006/2022.10(4).852.860
- El'kina GYa. Content of amino acids in plants at different levels of lead in the soil. Agrohimia. 2023;(6):63–72. (In Russ.). https://elibrary.ru/QOXLOQ
- Tabalenkona GN, Silina EV. Influence of habitat conditions on the content and composition of free amino acids of Plantago media L. leaves. Proceedings of Voronezh State University. Series: Chemistry. Biology. Pharmacy. 2023;(2):54–61. (In Russ.). https://elibrary.ru/WSFRVA
- Lezhnina MG, Khanina MA, Podolina EA, Rodin AP. The chemical composition of urban gravilate (Geum urbanum L.). The Prospects for Innovative Technologies in Medicine and Pharmacy: Proceedings of the 6th All-Russian Scientific and Practical Conference. Volume 2; 2019; Orekhovo-Zuevo. Orekhovo-Zuevo: State University of Humanities and Technology; 2019. p. 161–167. (In Russ.). https://elibrary.ru/DHIOFC
- Prosekov AYu, Ulrikh EV, Kozlova OV, Dyshlyuk LS. Study pectin as vegetable analog pharmaceutical gelatine. Modern Problems of Science and Education. 2014;(5). (In Russ.). https://elibrary.ru/SZVKBJ
- Boyarskykh IG, Siromlya TI. Macro- and trace elements composition of some medicinal plants in the geochemically abnormal environment in the Altai Mountains (Russia). Rastitelnye Resursy. 2022;58(4):376–387. https://doi.org/10.31857/S0033994622040045
- Yermokhin YuI, Bobrenko IA, Bobrenko EG. Microelement composition of agricultural plants in Siberia. Research and Scientific Electronic Journal of Omsk SAU. 2020;21(2).
- Kruglov DS, Prokusheva DL. The trace-element constituents of the most widespread plants of genus Artemisia. Chemistry of Plant Raw Material. 2022;(3):139–149. (In Russ.). https://doi.org/10.14258/jcprm.20220310800
- Hilfiker A, Bovet L. Modulation of the content of amino acids in the plant. Russia patent RU 2799785C2. 2023. https://elibrary.ru/DXYVMO
- Saleh TA. Trends in the sample preparation and analysis of nanomaterials as environmental contaminants. Trends in Environmental Analytical Chemistry. 2020;28. https://doi.org/10.1016/j.teac.2020.e00101
- Babich O, Sukhikh S, Pungin A, Asyakina L, Ivanova S, Prosekov A. Modern trends in the in vitro production and use of callus, suspension cells and root cultures of medicinal plants. Molecules. 2020;25(24). https://doi.org/10.3390/molecules25245805
- Babich O, Sukhikh S, Prosekov A, Asyakina L, Ivanova S. Medicinal plants to strengthen immunity during a pandemic. Pharmaceuticals. 2020;13(10). https://doi.org/10.3390/ph13100313
- Polozhij AV, Vydrina SN, Kurbatskij VI, Nikiforova OD. Flora Sibiriae. Tomus 9. Fabaceae (Leguminosae). Novosibirsk: Nauka; 1994. 280 p. (In Russ.).
- Popova E. Distribution of heavy metals in plant communities of the West Siberian Arctic and Subarctic. E3S Web of Conferences. 2021;284. https://doi.org/10.1051/e3sconf/202128401009
- Sardans J, Peñuelas J. Potassium control of plant functions: Ecological and agricultural implications. Plants. 2021;10(2). https://doi.org/10.3390/plants10020419
- Dyshlyuk L, Babich O, Prosekov A, Ivanova S, Pavsky V, Chaplygina T. The effect of postharvest ultraviolet irradiation on the content of antioxidant compounds and the activity of antioxidant enzymes in tomato. Heliyon. 2020;6(1). https://doi.org/10.1016/j.heliyon.2020.e03288
- Dolganyuk V, Sukhikh S, Kalashnikova O, Ivanova S, Kashirskikh E, Prosekov A, et al. Food proteins: Potential resources. Sustainability. 2023;15(7). https://doi.org/10.3390/su15075863
- D'yakova N A. Peculiarities of heavy metal and arsenic trans-medium transition along the chain “soil – medicinal plant material – water etracts”. Humans and their Health. 2023;26(1):64–71. (In Russ.). https://doi.org/10.21626/vestnik/2023-1/08
- Rubanka EV, Terletskaya VA, Zinchenko IN. The migration of heavy metals during the extraction of plant materials. Food Science and Technology. 2013;24(3):70–72. (In Russ.). https://elibrary.ru/XIKWEH
- Dmitrieva AI, Belashova OV, Ivanova SA, Prosekov AYu, Milenteva IS. Assessment of the content of heavy metals in medicinal plants of Genus trifolium from the growing area on the example of the Siberian federal district. International Journal of Pharmaceutical Research. 2020;12(3):1880–1893. http://dx.doi.org/10.31838/ijpr/2020.12.03.262
- Reut AA. The content of biologically active substances to the introduced members of the genus Hemerocallis L. News of FSVC. 2019;(1):93–96. (In Russ.). https://doi.org/10.18619/2658-4832-2019-1-93-96
- Faskhutdinova ER, Sukhikh AS, Le VM, Minina VI, Khelef MEA, Loseva AI. Effects of bioactive substances isolated from Siberian medicinal plants on the lifespan of Caenorhabditis elegans. Foods and Raw Materials. 2022;10(2):340–352. https://doi.org/10.21603/2308-4057-2022-2-544
- Kamanina IZ, Kaplina SP, Salikhova FS. The content of heavy metals in medicinal plants. Scientific Review. Biological Science. 2019;(1):29–34. (In Russ.). https://elibrary.ru/PQBWFP
- Kutlimurotova RKh, Pulatova LT. Study of the amino acid composition of Asarum europaeum L plants growing in Uzbekistan. Universum: Chemistry and Biology. 2021;86(8):27–30. (In Russ.). https://doi.org/10.32743/UniChem.2021.86.8.12131
- Babich OO, Milentyeva IS, Dyshlyuk LS, Ostapova EV, Altshuler OG. Structure and properties of antimicrobial peptides produced by antagonist microorganisms isolated from Siberian natural objects. Foods and Raw Materials. 2022;10(1):27–39. https://doi.org/10.21603/2308-4057-2022-1-27-39
- Dobrosmyslova IA, Sazanova AA, Semenov VG, Tuleubaev Zh, Yesimbekova ZT, Ziyaeva GK. Plant growth and biological productivity after processing with microelements. The Bulletin the National Academy of Sciences of the Republic of Kazakhstan. 2021;(1):74–80. (In Russ.). https://doi.org/10.32014/2021.2518-1467.10
- Prosekov AYu, Babich OO, Zaushintseva AV, Belashova OV, Milenteva IS, Asyakina LK, et al. Method for producing curd mass enriched with common skullcap and red clover concentrates. Russia patent RU 2753361C1. 2021. https://elibrary.ru/DIGWCS
- The State Pharmacopoeia of the Russian Federation. Vol. IV. Moscow; 2018. 1926 p. (In Russ.).