Аннотация
The Arctic is currently a strategic region of Russia and it requires military protection. Good nutrition is a prerequisite for successful performance of the servicemen in such extreme conditions. The Russian armed forces do not have a special ration for the Arctic region. The existing rations lack products enriched with biologically active substances, probiotics, prebiotics, or vitamin and mineral complexes. Subcaloric nutrition, even with short-term physical exertion, in combination with low temperatures deprives the human body of vitamins and minerals. Therefore, the development of freeze-dried bioproducts with functional properties is highly relevant. This article presents the results of a study aimed to develop new freeze-dried milk-based bioproducts for the Russian military in the Arctic. For this, we created a microbial consortium of lactic acid bacteria with a wide spectrum of antimicrobial activity. We also used a range of functional ingredients, namely pectin, pine nut meal, a ‘Lactobel’ prebiotic protein-and-carbohydrate product, rowan puree, and rosehip syrup in the amounts established during the study. The new freeze-dried milk-based bioproducts named ‘Pobeda’, enriched with combiotics and metabiotics, will provide the Russian military with better nutrition in the extreme conditions of the Arctic.Ключевые слова
Military nutrition in the Arctic, biotechnology, freeze-dried milk-based bioproducts, probiotic bacteria consortium, synbiotics, combiotics, metabioticsВВЕДЕНИЕ
Gaining control over the Arctic region is currently becoming not only a matter of geopolitics, but also a matter of survival in the future multipolar world. Its geographical and transport conditions, as well as ample energy resources, make the Arctic zone of Russia an area of increased interest not only for the Arctic states, such as the USA, Norway, Canada, and Denmark, but also for some non-Arctic countries, such as Japan, China, South Korea, and others. Therefore, this strategic region requires Russian military presence to protect the northern state border and ensure national security. Good nutrition of the Russian military is a prerequisite for the successful implementation of their duty in the extreme Arctic conditions [1–4].
In the Arctic, the human body is affected by a complex of adverse factors even if living conditions are quite satisfactory. These factors disturb almost all types of metabolism and greatly reduce the content of vitamins and glucose in the blood. According to scientists, even short-term physical exertion in combination with low temperatures and a subcaloric diet can lead to a deficiency of vitamins (C and group B), as well as macro- and microelements (potassium, calcium, magnesium, fluorine, selenium, and iodine). Moreover, low temperatures disturb the metabolism of vitamin C and the B vitamins [5–8, 34].
Currently, there are no special rations for the Russian military serving in the Arctic zone. Their diet is regulated by the Decree of the RF Government of December 29, 2007 ‘On food supplies for servicemen and other categories of staff, as well as feed (food) supplies for animals in military units and organisations in peacetime’. According to this decree, food rations for the military are as follows: ration no. 1 (common ration) with extra products for units in the Extreme North (canned fish 40 g, butter 15 g, biscuits 40 g, and condensed milk 25 g); ration no. 2 (summer ration); and ration no. 5 (medical ration) [9]. During the cold season (from October 1 to March 31), 100 g of rye and wheat bread is replaced with 20 g of salo (cured fatback) [10]. Thus, the limited range of products and a complete replacement of fresh fruits and vegetables with canned ones, cannot provide the Arctic servicemen with adequate nutrition.
The current system of army rations is based on the theory of sensible nutrition. This explains why they lack foods enriched with biologically active substances, eubiotics, prebiotics, vitamin-and-mineral complexes, and phytoprotectors. Therefore, their inclusion in the rations is perceived as an innovation.
The theory of functional nutrition is better suited for the Arctic conditions. Functional nutrition contains a variety of essential elements such as:
– fat-soluble and water-soluble vitamins (A, D, E, B1, B2, B6, B12, B9, C, and PP);
– vitamin-like substances (choline, L-carnitine, ubiquinone, lipoic and orotic acids);
– essential amino acids;
– polyunsaturated fatty acids (omega-3, omega-6);
– phospholipids;
– antioxidants (vitamins C, E, carotenoids);
– macro- and microelements (calcium, magnesium, potassium, iodine, selenium, iron, zinc, etc.);
– probiotics (probiotic microorganisms);
– prebiotics (dietary fibres, carbon-containing compounds of microbial and non-microbial origin, plant and microbial polysaccharides, etc.);
– synbiotics (probiotics + prebiotics);
– combiotics (synbiotics + functional ingredients, such as vitamin-and-mineral premixes, phenol-based and other plant compounds); and
– metabiotics (containing active metabolites, metabolic products of probiotic cultures) [3, 8, 11–13].
The Military Medical Academy, the RF Ministry of Defence is currently developing nutrition standards for military personnel serving in the Extreme North. They may include foods from local raw materials, such as plants (cranberries, lingonberries, blueberries, etc.), algae, mushrooms, and lichens [14].
The priority here is to develop various freeze-dried bioproducts, since they have a low weight, a small size, and a long shelf life. They also ensure the vitality of vitamins and probiotic microorganisms at low temperatures. Freeze-dried bioproducts are especially important as a survival ration for military personnel serving in remote areas, away from logistics bases, as well as for raid groups and special forces whose work involves increased physical activity and long-distance transitions. This makes the development of milk-based freeze-dried functional bioproducts a promising direction.
Thus, we aimed to develop a biotechnology for producing milk-based bioproducts with functional ingredients to improve the diet of servicemen in the Arctic.
ОБЪЕКТЫ И МЕТОДЫ ИССЛЕДОВАНИЯ
The study objects were Siberian starter cultures of lactic acid bacteria with a high content of viable cells, not less than 109 CFU/cm3. The cultures were adapted for Russians and had proven health-benefitting effects. They were:
– Lactobacillus acidophilus (L-1), Lactobacillus casei (L-2), and Lactobacillus delbrukii subsp. bulgaricus (L-4) produced by ZAO Vektor-BiAlgam, Novosibirsk;
– Lactococcus lactis subsp. lactis, Lactococcus lactis subsp. cremoris, Lactococcus lactis subsp. diacetilactis, and Streptococcus salivarius thermophilus (BK-Altai-Snzh) produced by the Barnaul Biofactory, Barnaul;
– their microbial consortium obtained during the study; and
– liquid and dry synbiotic bioproducts developed during the study.
Titratable acidity was determined according to State Standard 3624-92 by titration with a 0.1N sodium hydroxide with phenolphthalein and expressed in Turner degrees (°T).
Аctive acidity was determined by a potentiometric method according to State Standard 32892-2014 on a SG8-ELK SevenGo pro pH-meter / ion meter (Mettler Toledo, Switzerland).
The quantity of lactic acid bacteria cells was measured by the method of limiting dilutions on a dense agar medium MRS according to State Standard 10444.11-89 and State Standard 10444.11-2013.
The morphology of the bacteria was studied by making preparations stained with methylene blue, followed by microscopy in an immersion system with a 100x objective lens using a MIKMED-5 binocular microscope and photographing with a LOMO TC-500 camera.
The concentration of exopolysaccharides (EPSs) was determined by the anthrone method [15]. The action of concentrated sulfuric acid on carbohydrates forms furfural, 5-methylfurfural, or 5-hydroxymethylfurfural. Reacting with anthrone, they produce intensely coloured green or blue-green products. During the experiment, 4 volumes of distilled water and 10 volumes of freshly prepared anthrone reagent were added to 1 volume of the culture liquid of the bioproduct. Тhe mixture was then incubated for 10 min at 1,000°C in a water bath. The concentration of EPSs was determined by spectrometry on a Unico-2800 single-beam scanning spectrometer at a wavelength of 620 nm. Various concentrations of glucose solutions were used as a standard.
The selection of starter cultures in the microbial consortium was based on the microorganisms’ probiotic properties and their ability to synthesise EPSs.
The antagonistic activity of starter cultures of lactic acid bacteria and the microbial consortium against pathogenic and potentially pathogenic microorganisms was determined by the method of serial dilutions. The mixed populations were compared with the growth of test cultures in a liquid nutrient medium, followed by inoculation in solid media [16]. E.coli, S.aureus, Pr.vulgaris, Ps.mirabilis, Kl.pheumoniae, Sh.flexneri, Sh.sonnei, and S.сottbus were used as test microorganisms.
Antibiotic resistance of starter cultures of lactic acid bacteria and the microbial consortium was measured by the method of serial dilutions in a liquid nutrient medium.
Physical and chemical indicators of bioproducts were studied by standard methods.
The solubility index of freeze-dried bioproducts was determined according to State Standard 30305.4-95.
The moisture content of freeze-dried bioproducts was determined on an OHAUS MB-35 moisture analyser.
Sensory characteristics were evaluated according to State Standard 28283-89 and State Standard R ISO 22935-2-2011.
Safety indicators of the bioproducts were assessed against the medical and biological requirements established by the regulatory documents [17].
The statistical processing of experimental data derived from 3–5 replications was performed with Statistica 12. The differences were considered significant with p < 0.05.
РЕЗУЛЬТАТЫ И ИХ ОБСУЖДЕНИЕ
Our earlier studies found that fermented milk products based on microbial consortia of lactic acid bacteria that synthesised exopolysaccharides had plenty of benefits. In addition to improved rheological properties, they had a wider spectrum of antimicrobial activity, compared to starter cultures. This promoted adhesion of beneficial probiotic bacteria to the intestinal walls and ensured maximum effectiveness for the human body.
Numerous studies by Khamagaeva et al. proved that multi-strain starters had a higher biotechnological potential and were more resistant to adverse environmental factors. Therefore, for this study, we created a consortium of lactic acid bacteria with the greatest ability to synthesise EPSs (Fig. 1) and a wide spectrum of antimicrobial activity.
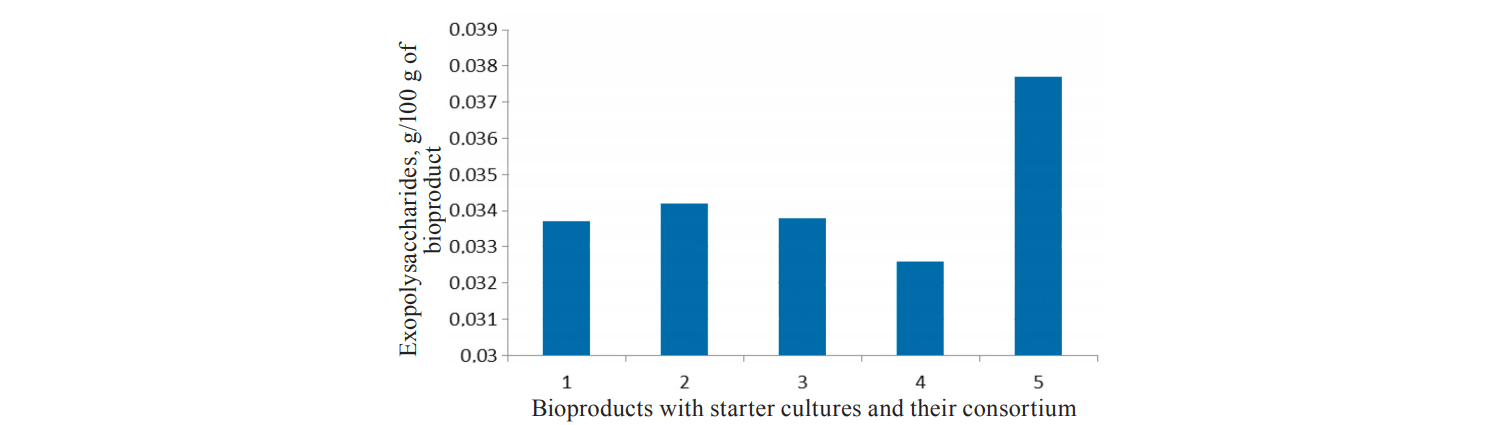
1 – bioproduct with Lactobacillus acidophilus (L-1), 2 – bioproduct with Lactobacillus delbrukii subsp. bulgaricus (L-2), 3 – bioproduct with Lactobacillus casei (L-4), 4 – bioproduct with Lactococcus lactis subsp. lactis, Lactococcus lactis subsp. cremoris, Lactococcus lactis subsp. diacetilactis, Streptococcus salivarius thermophilus (BK-Altai-Snzh), 5 – bioproduct with the microbial consortium (1:2:3:4 in the ratio of 1:1:0.8:1.2).
The synthesis of EPSs usually depends on the composition of the microbial consortium and the conditions of its cultivation. For example, an increase or decrease in the optimal growth temperature causes an active synthesis of EPSs. This was confirmed by our studies with a cultivation temperature of 38 ± 1°С for bioproducts with the consortium. This cultivation temperature was not optimal for all the lactic acid bacteria making up the microbial consortium.
In addition, a greater synthesis of EPSs leads to closer intercellular contacts between rod-shaped and coccoid microorganisms in the microbial consortium. Fig. 2 shows the morphology of microflora in bioproducts with starter cultures and their microbial consortium.
Increased formation of EPSs makes the bioproduct more viscous and enhances cohesion (aggregation). As shown in studies [18–21], cohesion is an accumulation of cells, a kind of a supra-organism system similar to a multicellular organism. It is a cooperation of individual cells whose concerted activity is aimed at achieving the same result. The exchange of information and signals in this system is carried out by extracellular metabolites that regulate the activity of bacteria. Such interactions ensure physiological and adaptive resistance of cells to negative environmental effects.
As can be seen in Fig. 2, the aggregation of microorganism colonies in the microbial consortium demonstrates their ability for multicellular organisation, which is characteristic of bacteria in the gastrointestinal tract. In addition, a bioproduct with a microbial consortium has a wider spectrum of antagonistic activity against pathogenic and potentially pathogenic microorganisms (Fig. 3), compared to the starter cultures present in it. Such products are also more resistant to various antibiotics (Table 1) and can therefore be used in antibiotic therapy.
As can be seen in Fig. 3, the microflora in the bioproduct with a microbial consortium displayed the highest antagonistic activity against E.coli, Pr.vulgaris and S.cottbus, with the number of viable cells being 102 CFU per 1 cm3. This was probably due to the ability of lactic acid bacteria to produce antimicrobial substances, such as nisin (Lactococcus lactis subsp. lactis), diplocin (Lactococcus lactis subsp. cremoris), bulgaricin (Lactobacillus delbrukii subsp. bulgaricus), and lactocins B, F, G, and M (Lactobacillus acidophilus).
Table 1 shows a greater resistance of microflora in bioproducts with a microbial consortium to all the antibiotics, compared to the starter cultures. This is probably due to the acquisition of new genetic information through the transfer of plasmids during conjugation and transduction by not only closely related bacterial species, but also by bacterial genera. Changes in the cultivation temperature appear to cause rearrangements in the lactococcal genome. Lactococcus plasmids are able to encode such properties as the production of antibiotic substances and the preservation of antibiotic resistance.
At the next stage, we studied the influence of various functional prebiotic substances on the growth of microflora in the microbial consortium of dairy bioproducts. Namely, we used pectin, ‘Lactobel’ (а milk protein-and-carbohydrate product), pine nut meal, ‘Sibirskaya’ rowan puree (developed by Kemerovo State University), and rosehip syrup. In the course of experimental studies, we established the most optimal process parameters and amounts of the above prebiotic substances for producing new bioproducts. The combined process flow chart is shown in Figure 4.
The biotechnology for producing freeze-dried products involves standard operations used in the production of liquid fermented dairy products [22]. After fermentation, the finished bioproduct is cooled and freeze-dried.
People can consume both liquid bioproducts and freeze-dried bioproducts in the form of tablets, highly water-soluble dry powders, or bio-sweets.
Combining dairy raw materials with plant components, we can create functional bioproducts with a balanced composition that have a health-benefitting effect and are better absorbed by the human body. Pectin was chosen as a polysaccharide with prebiotic properties that is present in all algae and land plants. It is a common stabilising and gelling agent used in the food industry to give products good rheological properties. Pectin is one of few natural organic compounds that does not undergo chemical degradation when passing through the acidic and alkaline enzymatic barriers of the human body. It maintains its chemical structure and properties in the gastrointestinal tract, helping to restore its mucous membranes. In addition, pectin normalises colonic motility, regulates intestinal microflora, inhibits putrefactive microflora, and participates in most of essential physiological processes. Therefore, most countries recognize pectin as a physiologically valuable functional ingredient that improves human health when present in conventional diets [23–29].
The choice of ‘Lactobel’, a Russian milk protein-and-carbohydrate product with prebiotic action, was determined by its properties. This slightly yellow powder with a sweetish taste of powdered milk is highly soluble in warm water and skim milk. Pasteurisation does not change its technological properties, i.e. it does not cause proteins to precipitate, coagulate, or denature. In addition, ‘Lactobel’ contains albumin, caseinates, lactose, whey proteins, and a prebiotic (lactulose). Its prebiotic and bifidogenic properties revitalise microflora in the microbial consortium and beneficial microflora in the human gastrointestinal tract. As a result, its use ensures a synbiotic bioproduct with highly nutritional and biological values.
The use of Russian pine nut meal creates a synbiotic dairy bioproduct with functional properties. According to some studies [30–32], pine nut meal is a granular powder with the taste of a pine nut that activates beneficial microflora in the gastrointestinal tract and has prebiotic and bifidogenic properties. Its protein composition includes 19 amino acids: tryptophan, leucine, isoleucine, valine, lysine, methionine, glutamic acid, histidine, proline, serine, glycine, threonine, alanine, aspartic acid, phenylalanine, cystine, cysteine, arginine, and tyrosine. Of these, 70% are essential and conditionally essential aminoacids, which indicates a high biological value of the protein. Pine nut meal proteins contain more methionine (up to 5.6 g/100 g of protein), cysteine and tryptophan (3.4 g/100 g of protein) than the ideal protein. Their digestibility (95%) is similar to that of chicken egg proteins.
The carbohydrate composition of pine nut meal includes polysaccharides (starch, fibre, pentosans, and dextrins) and water-soluble sugars (glucose, fructose, sucrose, and raffinose). A high content of dietary fibre gives pine nut meal prebiotic properties. Its vitamin composition includes mainly fat-soluble vitamins (A, E, D, K, and F), group B vitamins, and folic acid. Its content of macro- and microelements makes pine nut meal a unique natural source of essential minerals that play an important role in many biochemical processes in the human body. It contains up to 5% of mineral substances such as copper, manganese, vanadium, potassium, phosphorus, calcium, molybdenum, nickel, iodine, boron, zinc, iron, phosphorus, and magnesium. Only 100 g of pine nut meal meets the daily human need for magnesium, manganese, copper, zinc, cobalt, and iodine. In addition, Siberian pine nut meal contains barium, titanium, silver, aluminium, iodides, and sodium. Its lipids are represented by phospholipids, cerebrosides, sulfolipids, and unsaturated fatty acids (oleic, linolenic, and gamma-linolenic). They give the bioproduct an attractive appearance, natural taste, smell, and aroma of the raw material, thus increasing its sensory characteristics.
Siberian rowan berries and rosehips were chosen due to their healing properties and ubiquity in Western and Eastern Siberia. Rowan berries contain a complex of important vitamins such as C, E, K, P (rutin), PP (niacin), B group (thiamine, riboflavin, and folic acid), vitamin A, and biologically active substances. They have a higher content of vitamin C (70 mg) than blackcurrant and lemon and are richer in carotene (vitamin A) than carrots. Rowan berries contain such minerals as magnesium (331 mg), potassium (323 mg), as well as calcium (58 mg), phosphorus (36 mg), and sodium (9 mg). Their trace elements include copper, manganese, iron, and zinc. They also contain organic acids (citric, tartaric, and ursolic), easily digestible sugars (sucrose, sorbose, fructose, and glucose), hemicellulose, fibre, and pectic substances. We developed a special technology to make rowan puree.
Russian rosehip syrup is a pleasant-tasting, sweet, viscous dark brown liquid rich in vitamins C and P, pectins, and flavonoids. It has a tonic effect, boosts immunity, prevents hypovitaminosis and malignant neoplasms, and restores energy in fatigued and physically exhausted people. Also, it enhances vision, improves the functioning of blood-making organs, and normalises blood pressure. Finally, rosehip syrup has powerful antioxidant properties, participates in the synthesis of collagen, prevents the destruction of bones, has a positive effect on the regeneration of tissues and bones in cases of injuries or fractures, and accelerates recovery from colds [33].
Table 2 shows the key quality indicators of new ‘Pobeda’ freeze-dried bioproducts developed during the study.
According to the data obtained during the study, the ‘Pobeda’ freeze-dried bioproducts have good sensory characteristics and contain a sufficient number of viable lactic acid bacteria cells. These new freeze-dried bioproducts can be produced in the existing production facilities, on fermented milk and freeze-dried biofoods production lines.
ВЫВОДЫ
The ‘Pobeda’ freeze-dried bioproducts of protein and fat orientation with combiotics and metabiotics can enrich the diet of the Russian military performing special tasks in the Arctic. They can also be used in rations of servicemen performing long-transition raids with increased physical activity and in survival rations.
КОНФЛИКТ ИНТЕРЕСОВ
The authors declare no conflict of interest.
СПИСОК ЛИТЕРАТУРЫ
- Prosekov A.Yu. and Ivanova S.A. Providing food security in the existing tendencies of population growth and political and economic instability in the world. Foods and Raw Materials, 2016, vol. 4, no. 2, pp. 201–211. DOI: https://doi. org/10.21179/2308-4057-2016-2-201-211.
- Osnovy gosudarstvennoy politiki Rossiyskoy Federatsii v Arktike na period do 2020 goda na dalʹneyshuyu perspek- tivu [The framework of the state policy of the Russian Federation in the Arctic for the period up to 2020]. Rossiyskaya gazeta [Rossiyskaya Gazeta], 2009, no. 4877, pp. 32. (In Russ.).
- Makov V.A. Rations specificatiobs for military personnel in the Arctic region. Russian Arctic, 2018, no. 3, pp. 51–59. (In Russ.).
- Prosekov A.Yu. and Ivanova S.A. Food security: The challenge of the present. Geoforum, 2018, vol. 91, pp. 73–77. DOI: https://doi.org/10.1016/j.geoforum.2018.02.030.
- Blaginin A.A., Vislov A.V., and Lizogub I.N. Actual questions of medical maintenance of aviation specialists in thearctic region. Voenno-meditsinskiy zhurnal [Military Medical Journal], 2015, vol. 336, no. 1, pp. 50–54. (In Russ.).
- Solonin Yu.G. and Boyko E.R. Medical and physiological aspects of vital activity in the Arctic. Arctic: Ecology and Economy, 2015, vol. 17, no. 1, pp. 70–75. (In Russ.).
- Krivtsov A.V., Kirichenko N.N., Ivchenko E.V., et al. Physiological and hygienic characteristics of food and wa- ter supply in military garrison in Arctic region. Vestnik of Russian military medical Academy, 2015, vol. 52, no. 4, pp. 165 – 168. (In Russ.).
- Larin L. and Shagarov D. VusloviyakhArktiki i Kraynego Severa [In the conditions of theArctic and the Extreme North]. Orientir [Orientir], 2016, no. 2, pp. 21–23. (In Russ.). Available at: http://stmzavod.ru/orientir_feb_%E2%84%962. pdf. (accessed 1 March 2019).
- Postanovlenie pravitelʹstva RF ot 29 dekabrya 2007 g. № 946 ‘O prodovolʹstvennom obespechenii voennosluzhash- chikh i nekotorykh drugikh kategoriy lits, a takzhe ob obespechenii kormami (produktami) shtatnykh zhivotnykh voinskikh chastey i organizatsiy v mirnoe vremya’ [The Decree of the RF Government No. 946 of December 29, 2007 ‘On food supplies for servicemen and other categories of staff, as well as feed (food) supplies for animals in military units and organisations in peacetime’]. Moscow, 2008. 80 p.
- Prikaz Ministra Oborony RF ot 21 iyunya 2011 g. № 888 ‘Ob utverzhdenii Rukovodstva po prodovolʹstvennomu obespecheniyu voennosluzhashchikh Vooruzhennykh sil Rossiyskoy Federatsii i nekotorykh drugikh kategoriy lits, a takzhe obespecheniyu kormami (produktami) i podstilochnymi materialami shtatnykh zhivotnykh voinskikh chastey v mirnoe vremya’ [The Order of the RF Minister of Defense No. 888 of June 21, 2011 ‘On the approval of the Guide- lines on food supplies for servicemen of the Armed Forces of the Russian Federation and some other categories of staff, as well as feed (food) and bedding supplies for animals in military units in peacetime’]. Moscow, 2011. 88 p.
- Shenderov B.A. Probiotics and Functional Foods. In: Encyclopedia of Life Support Systems (EOLSS). Oxford, UK: Eolss Publ., 2011.
- Ardatskaya M.D. Probiotics, prebiotics and metabiotics in the management of microecological bowel disorders. Med- ical Council, 2015, no. 13, pp. 94–99. (In Russ.).
- Chervinets Yu.V., Chervinets V.M., and Shenderov B.A. Modern concept on the biotechnological potential of symbi- otic human microbiota. Volga Medical Journal, 2018, vol. 17, no. 1, pp. 19–26. (In Russ.).
- Nikitenko M.E. and Trofimova I.B. Food decurity in the Arctic territories of the Russian Federation. Society: Politics, Economics, Law, 2016, no. 9. (In Russ.).
- Piskaeva A.I., Babich O.O., Dolganyuk V.F., and Garmashov S.Y. Analysis of influence of biohumus on the basis of consortium of effective microorganisms on the productivity of winter wheat. Foods and Raw Materials, 2017, vol. 5, no. 1, pp. 90–99. DOI: https://doi.org/10.21179/2308-4057-2017-1-90-99.
- Pirog T.P., Grinberg T.A., and Malashenko Yu.R. Protective functions of exopolysaccharides produced by an Acineto- bacter sp. Microbiology, 1997, vol. 66, no. 3, pp. 279–283.
- TR TS 033/2013. O bezopasnosti moloka i molochnykh produktov [Technical Regulations of the Customs Union033/2013. On milk and dairy products safety].
- Vorob’eva L.I. Stressors, stress reactions, and survival of bacteria: A review. Applied Biochemistry and Microbiology, 2004, vol. 40, no. 3, pp. 261–269. (In Russ.).
- Lisitskaya K.V., Nikolaev I.V., Torkova A.A., Popov V.O., and Koroleva O.V. Analysis of functional properties of biologically active substances using eukaryotic cell models (review). Applied Biochemistry and Microbiology, 2012, vol. 48, no. 6, pp. 581–598. (In Russ.).
- Nikolaev Yu.A. Extracellular factors of bacterial adaptation to unfavorable environmental conditions. Applied Bio- chemistry and Microbiology, 2004, vol. 40, no. 4, pp. 387–397. (In Russ.).
- Magdanova L.A. and Golyasnaya N.V. Heterogeneity as an adaptive trait of microbial populations. Microbiology, 2013, vol. 82, no. 1, pp. 3–13. (In Russ.).
- Prosekov A.Yu. Teoreticheskoe obosnovanie i tekhnologicheskie printsipy formirovaniya molochnykh penoobra- znykh dispersnykh sistem. Diss. dokt. tekhn. nauk [Theoretical substantiation and technological principles of the formation of dairy foam dispersed systems. Dr. eng. sci. diss]. Kemerovo, 2004. 42 p.
- Valyshev A.V. and Golovchenko V.V. Prebiotic activity of pectins and their derivatives. Byulletenʹ Orenburgskogo nauchnogo tsentra UrO RAN (ehlektronnyy zhurnal) [The Bulletin of the Orenburg Scientific Centre, the Ural Branch of the Russian Academy of Sciences (electronic journal)], 2012, no. 3. (In Russ.).
- Yeo S.K. and Liong M.T. Effect of prebiotics on viability and growth characteristics of probiotics in soymilk. Journal of the Science of Food and Agriculture, 2010, vol. 90, no. 2, pp. 267–275. DOI: https://doi.org/10.1002/jsfa.3808.
- Dyshlyuk L., Babich O., Belova D., and Prosekov A. Comparative Analysis of Physical and Chemical Properties of Biodegradable Edible Films of Various Compositions. Journal of Food Process Engineering, 2017, vol. 40, no. 1. DOI: https://doi.org/10.1111/jfpe.12331.
- Shenderov B.A. Meditsinskaya mikrobnaya ehkologiya i funktsionalʹnoe pitanie. T. 1: Mikroflora cheloveka i ee funktsii [Medical microbial ecology and functional nutrition. Vol. I: Human microflora and its functions]. Moscow: Grant Publ., 1998. 287 p. (In Russ.).
- Shenderov B.A. Meditsinskaya mikrobnaya ehkologiya i funktsionalʹnoe pitanie. T. 3: Probiotiki i funktsionalʹnoe pitanie [Medical microbial ecology and functional nutrition. Vol. III: Probiotics and functional nutrition]. Moscow: Grant Publ., 2001. 288 p. (In Russ.).
- Shenderov B.A. Funktsionalʹnoe pitanie [Functional nutrition]. Moscow: Gran Publ., 2002. 296 p. (In Russ.).
- Lisitsyn A., Kuznetsova O., Minaev M., and Prosekov A. Studying of Lactulose Hygroscopicity and Microstructure after Spray Dehydration. Research Journal of Pharmaceutical Biological and Chemical Sciences, 2016, vol. 7, no. 2, pp. 883–890.
- Hamagaeva I.S. and Kaluzhskih Yu.G. Effects of vegetable raw materials on consuming properties of bioproducts. Dairy Industry, 2009, no. 7, pp. 38. (In Russ.).
- Khanturgaev A.G. Bifidobacteria cultivation in milk with cedar oilcake. ESSUTM Bulletin, 2013, vol. 45, no. 6, pp. 117–122. (In Russ.).
- Shiretorova V.G. Mineral composition of siberian pine seeds and its products. ESSUTM Bulletin, 2014, vol. 46, no. 1, pp. 93–96. (In Russ.).
- Zhurba O.V. and Dmitriev M.Ya. Lekarstvennye, yadovitye i vrednye rasteniya [Medicinal, poisonous and harmful plants]. Moscow: KolosS Publ., 2008. 512 p. (In Russ.).
- Tien N.P., Siripongvutikorn S., and Usawakesmanee W. Prototype of Vietnamese tamarind fish sauce fortified with iron, zinc and vitamin A. Foods and Raw Materials, 2018, vol. 6, no. 1, pp. 110–119. DOI: https://doi.org/10.21603/2308- 4057-2018-1-110-119.