Аннотация
The potentials of rice bran sourdough in bread making are recently gaining popularity. However, there is no information on the influence of processing conditions on the quality attributes of rice bran sourdough. To inves- tigate the influence of fermentation time and temperature on the levels of acidity (pH and TTA) in rice bran sour-dough fermented with L. plantarum, we applied response surface methodology (RSM). Furthermore, we studied the effect of different fermentation time and temperature on the total phenolic and volatile compounds in the sourdough. GC/MS measurements for the evolution of aroma volatile compounds (VOCs) in the rice bran sourdoughs were conducted. The higher and longer the fermentation temperature and time, the higher the acidity levels in the sour- doughs. Fermentation temperature and time do not have a significant effect on the total phenolic sourdough con- tents. Forty-seven VOCs were detected in the rice bran sourdoughs. The major VOCs were acetic acids, ethanol, 2-Methoxy-4-vinylphenol, Hexadecanoic acid, 1-(hydroxymethyl)-1,2-ethanediyl ester, acetoin, and 2-methoxy-Phe- nol. The sourdough fermented at 35°C for 13 ho contained the largest number (27) of aroma compounds and had the highest acidity. These fermentation conditions are close to the optimal parameters (temperature – 33°C, duration – 12.5 hours), obtained as a result of applying RSM for rice bran fermentation. Thus, high quality bran sourdough can be produced at the temperature of 33°C for 12.5 hours. The results of this study will be useful to produce a quality rice bran sourdough bread with appealing aroma and a long shelf-life.Ключевые слова
Rice bran, acidity, Response surface methodology, Lactobacillus plantarum, HS-SPME, GC/MS, volatile compoundsВВЕДЕНИЕ
Rice bran is a by-product of rice milling industries, grossly underutilised in rice-producing countries. Although, rice bran is rich in dietary fibre, amino acids, minerals, and other bioactive compounds, which can have a beneficial effect on human health [1], it is commonly used as a livestock feed as opposed to human food formulation. This is because the use of bran in food industries, especially in baked goods, poses several technological problems, such as weakening of wheat dough structure and of baking quality, the decrease in bread volume and elasticity of bread crumb [2]. Bio-processing technologies, such as enzymatic and fermentation processes have received more attention recently due to their potential to improve nutritional and technological functionality of bran [3]. These processes have been employed for bran sourdough production [4–6].
Sourdough is used as a starter for bread making, because of its ability to improve bread quality [7, 8]. Traditionally, bread volume, crumb texture, flavour, and shelf-life of bread are enhanced using sourdough fermentation [2, 9, 10]. This is because baked products with sourdough are better protected from bacterial and mould spoilage, and have extended shelf-life [11, 12]. However, the preservative effect of sourdough depends on the bacteria used for the dough fermentation. Previous studies have reported an extended shelf life for sourdough bread produced with lactic acid bacteria (LAB), fermented rice bran sourdough [13, 14].
The flavour of baked foods, especially bread, is influenced by the recipe, processing condition, and volatile organic compounds produced by a starter culture. The generation of odorants in sourdough occurs due to enzymatic and microbial processes during sourdough fermentation [15], for example, LAB is responsible for liberation of aroma precursors and acidification in sourdough [7].
Fermentation temperature and time are important processing factors to be considered in producing a quality sourdough. The extent of metabolic performances of microorganisms in sourdough depends directly on the fermentation time, while fermentation temperature plays an important role in the stability of sourdough ecosystem [16, 17]. There have been studies on the effect of processing conditions and starter cultures on the formation of volatile compounds in wheat sourdoughs [18]. Volatile compounds produced by different species of lactobacilli in rye sourdough have also been reported [7]. There is little information on the optimisation of fermentation conditions for rice bran sourdough production, and no information on volatile compounds in LAB fermented rice bran sourdough.
Response Surface Methodology (RSM) is a multivariate technique used for an analytical optimisation [19]. In recent years, RSM has been used to optimise fermentation conditions for cereal bran. Application of RSM for optimisation of rice bran fermentation conditions for protein concentrate extraction, using baker’s yeast, has been studied [20]. RSM was also applied to study fermentation effects on the levels of phytochemicals in rye bran [5].
The use of rice bran sourdough is gaining popularity in bread making [13, 14, 21]. It is therefore important to establish optimum fermentation temperature and time for quality rice bran sourdough preparation. Furthermore, bread quality mostly depends on taste and aroma. Apart from aroma development during baking, aroma precursors are also produced during fermentation process. Volatile organic compounds (VOCs) in fermented sourdough contribute to bread flavour [22] and are produced from enzymatic, or autoxidation of flour lipids, microbial, yeast metabolism, and Maillard reaction [15]. However, publications about VOCs in sourdough are mostly about wheat, wheat germ, buckwheat, rye, rice, maize, Kefir or chestnut-flour sourdough using either GC-MS, or HRGC-MS [15].
Although, VOCs of rice bran oil have been characterised by GC-MS [23], to our knowledge, there has been no study on the characterisation of VOCs in rice bran sourdough using HS-SPME method. The objectives of this study were to optimise important fermentation factors (temperature and time) for rice bran sourdough production using RSM and to determine the effect of fermentation conditions on pH, titratable acidity (TTA), and total phenolics in L. plantarum fermented rice bran sourdough. For the first time, VOCs profile of rice bran sourdough samples fermented at different temperature and time was also identified and quantified by SPME-GC/MS analysis.
ОБЪЕКТЫ И МЕТОДЫ ИССЛЕДОВАНИЯ
Raw materials. Rice variety MR bran 219 was obtained from Padiberas Nasional Berhad (BERNAS) rice mill, Sekinchan, Selangor, Malaysia. The rice bran contained 7.2% of moisture, 11.7% protein, 18% fat, 8.6% fibre, 8.5% ash, and 45.9% carbohydrate, as determined in our previous study [21]. The bran was sieved using a Haver EML digital plus test sieve shaker (Harver and Boecker, 59302, OELDE, Germany) to particle size of < 300 µm.
Microbial strain. Lactobacillus plantarum. ATCC® 8014™ was purchased from the American Type Culture Collection (ATCC) (USA). SupelcoSPME fibre coated with 85-µm of Carboxen–polydimethylsiloxane (Carboxen/PDMS) was purchased from Bellefonte, PA (USA), SPME vials were purchased from LA-PHA-PACK (Germany). Distilled deionized water from Sartorius, Sdn, Bhd (Malaysia) was used for all sample preparations. N-alkanes (C8–C20), and 4-methyl-2-pentanol were purchased from Sigma-Aldrich (St Louis, USA). All other chemicals and reagents were of analytical grade.
Optimisation of rice bran fermentation conditions. We used RSM to investigate the combined effects of significant variables (fermentation temperature and time) in the production of rice bran sourdough. The adopted experimental design was a central composite design (CCD). The minimum and maximum independent variable levels were selected on the basis of previous sourdough studies [18, 5]. The CCD was divided into three blocks to calculate the reproducibility of the method. The CCD’s use required 13 runs for each block and the total of 39 runs for the whole design. Table 1 shows the experimental runs in the order they were performed. The dependent/response variables for the rice bran sourdough quality were pH and total titratable acidity (TTA). The independent variable range was 20–35°C of fermentation temperature and 6–20 hours of fermentation time. For reproducibility, the experiments were carried out in triplicate. For each of the response variable, a model summary, and a lack of fit test were constructed for linear, square, interaction and quadratic models. We investigated the behaviour of the response surface for the response function (Yp, predicted response) using a regression polynomial equation. The generalised polynomial mode to predict the response variable was:
where β0 was intercept, β1 and β2 were coefficients. The most accurate model was chosen based on a number of significant terms (p < 0.05), percentage of regression, the lack of fit test, and P-value for a regression model. A three-dimensional response surface plot was generated for each response variable. The reliability of the model was evaluated from the regression of the model (R2) and predictive power of the model (Q2). Generally, for a good fit model R2 should be ≥ 0.8 [24], however, the models with R2 and Q2 values of ≥ 0.9 are considered excellent.
Optimal processing conditions to determine rice bran sourdough production were calculated by performing a multiple response method called desirability. The desires and priorities for each of the variables were incorporated into the optimisation method. pH and titratable acidity (TTA) were chosen as the two most important quality parameters in this study, because sourdough quality depended mainly on them [25]. Since a rice bran falls within a high extraction rate category with 95% extraction rate, the desired pH target was in the range of 3.5 to 4.5 [26], while the desired TTA was in the range of 16 to 22 ml NaOH [26, 27].
Microbial strain and preparation of sourdough culture. Lactobacillus plantarum media were prepared according to a manufacturer’s instruction and sterilised by autoclaving at 121°C for 15 min. L. plantarum was the culture of De Man Rogosa and Sharpe (MRS) broth at 37°C for 72 hours at the atmosphere of 5% CO2. 1 ml of cultured MRS broth was mixed with 9 ml of sterile peptone water 0.1% (v/v) and diluted in ten-folds using serial dilution (10–2 to 10–10). A serial suspension (0.1 ml) was incubated on a plate count agar, and the colonies were counted. The bacteria suspension (109 CFU/ml) in peptone water (0.1%) was used for bran fermentation.
Preparation of rice bran sourdough. Rice bran sourdough was prepared according to [21]. 100g of rice bran were mixed with 130 g of distilled deionised water and 15 ml of L. Plantarum suspension (109 CFU/ml) in a sterilised beaker. Then the mixture was covered with aluminium foil and allowed to ferment in a fermenting box (Model Fx-11, Good and Well, SDN BHD, Malaysia) to produce sourdough. Fermentation temperature and time were in accordance with the experimental design of Table 1. The sourdough samples were frozen at –20°C until further analysis. Rice bran that was allowed to ferment naturally was used as a control.
pH and total titratable acidity (TTA) of rice bran sourdough. pH and TTA were measured as described by [25]. Frozen rice bran sourdough samples were thawed overnight in a refrigerator at 4°C. 10 g of the thawed sourdough were mixed with 100 ml of distilled water to obtain a suspension. The pH value of the suspension was measured by using a pH meter. TTA was determined by titrating the suspension against 0.1M NaOH to a final pH value of 8.5. TTA was expressed as the amount of NaOH (ml) used for titration and as a mean value of three replicates.
Total phenolic compound (TPC) analysis. A modified method of [28] was used for a total phenolic compound determination. 200 mg of the rice bran sourdough fermented with L. plantarum at different temperatures and time were extracted with 4 ml of 70% aqueous acetone by shaking in a rotary shaker (260 rpm, 2 hours) at room temperature. The supernatant liquid was collected after centrifugation (Hereaus Instruments D867 bench top centrifuge, Germany) at 3,000 g for 10 min. 200 µl of the supernatant were added to 1.5 ml of freshly prepared Folin Ciocalteu reagent (1:10 v/v with water). After 5 min equilibration, 1.5 ml of the sodium carbonate (60 g/l) solution was added to the mixture. The mixture was incubated at room temperature for 90 min. The absorbance of the mixture was read at 725 nm using 70% aqueous acetone as blank. The gallic acid calibration curve (0 to 1,000 µg/ml) was plotted, and TPC was expressed as micrograms (µg) of gallic acid equivalents (GAE) per gram of rice bran sourdough.
Characterisation of volatile compounds (VOCs) in rice bran sourdough. Volatile acids are generally produced, when cereals are fermented by lactic acid bacteria, such as L. plantarum. Volatile acids of cereal dough, such as rye dough and baked product (bread), are reported to be acetic and lactic acids. These acids are responsible for an acidic bread flavor [29].
Headspace solid phase microextraction (HS-SPME) analysis. The volatile fraction of rice bran sourdough samples was analysed by headspace sampling using solid phase microextraction (SPME) method described by [30], with some modifications. The VOCs of rice bran sourdoughs fermented with L. plantarum at different temperature and time (Table 2) were identified and quantified. 2 g of a sourdough sample fermented at different temperature and time (Table 2, sample A–J) were placed in a 20 ml headspace vial. 2 ml of a distilled deionised water and 5 µl of 4-methyl-2-pentanol (internal standard, 100 mg/l) were added to the headspace vial. The vial was placed in a thermostatic block on a stirrer at 60°C, then the fibre was inserted and maintained in the sample head space for 30 min, after which it was removed and immediately inserted into the GC/MS injector port for the desorption of compounds. A silica fibre coated with 85-µm of Carboxen–polydimethylsiloxane (Carboxen/PDMS) was used for the analyses. The fibre was conditioned prior to its first use. The internal standard (4-methyl-2-pentanol) was used for all analyses to monitor the SPME extraction and fibre performance during the analysis, as well as for semi-quantitative analysis of VOCs.
Gas chromatography–mass spectrometry (GC/MS) analysis and VOCs identification. The method described by [31] and [30] was adopted for the GC/MS analysis. The VOCs of the sourdough samples were determined with a gas chromatograph and a mass spectrometer equipped with a 30 m, 0.25 mm ID, film thickness of 0.25 µm, and capillary column (TG-WAXMS, Thermo Scientific, USA). The gas carrier was helium with a flow of 1.5 ml/min. SPME injections were splitless (straight glass line, 0.75 mm ID) at 240°C for 20 min (during which time thermal desorption of analytes from the fibre occurred). The oven parameters were as follows: the initial temperature was 40°C held for 3 min, followed by an increase to 240°C at a rate of 5°C /min, then held for 10 min. A mass spectrometer was operated in a scan mode from m/z 33–300 (2 s/scan) at an ionisation potential of 70 eV.
Identification of volatile compounds was achieved by comparing their mass spectra with the reference mass spectra library (NIST, version 2.0) and by matching the retention indices (RI) calculated according to the equation of [32], based on a series of alkanes, ranging from n-octane to n-eicosane (extrapolation using C18–C20 was used for compounds eluting after C20). A semi-quantitative analysis was also obtained by comparison of the VOCs peak areas with that of the internal standard obtained from the total ion chromatograms, using a response factor. Blank of the fibre and blank of the empty vial were conducted after every 10 analyses. All the analyses were performed in replicates.
Statistical analysis. The experimental design and optimisation procedure were performed using Minitab® version 16.0 software (Pennsylvania, USA). The data obtained were subjected to one way analysis of variance (ANOVA) to determine regression coefficients and a statistical significance of model terms to fit the mathematical models to the experimental data. Significant differences in the total phenolic compounds were compared by means of Duncan’s multiple comparison tests at 95% confidence level (p ≤ 0.05). The data were reported as mean values of replicate analyses.
РЕЗУЛЬТАТЫ И ИХ ОБСУЖДЕНИЕ
Influence of fermentation conditions on pH, and titratable acidity (TTA). The pH and TTA of the rice bran before fermentation (control) was 6.11 and 11.89, respectively (the results are not shown). The acidity levels (pH and TTA) of the rice bran sourdough fermented at different times and temperatures are presented in Fig. 1. The pH of the sourdough decreases with increasing fermentation time and temperature, whereas the TTA values increase.
The pH of the rice bran sourdough ranged from 4.0 to 4.6, while the TTA ranged from 13.9 to 23.4 ml of 0.1M NaOH/10 g sourdough (Fig. 1). Fermentation time was reported to be the most important parameter of wheat sourdough acidity, fermented with a single or combined starter of lactobacilli or yeast [18]. In contrary, the findings of this study showed that fermentation temperature and time were the most influential parameters of L. plantarum fermented rice bran sourdough acidity. The most acidic rice bran sourdough was the sample, fermented at 35°C for 13 hours, while the least acidic sourdough was the sample, fermented at 20°C for 13 hours (Fig. 1). This result indicates that there was a strong interaction between fermentation time and temperature during sourdough fermentation. The maximum TTA and the minimum pH values were obtained in the sourdoughs, fermented at 35°C for 13 hours. This is probably because at the optimum proliferation temperature (37°C, which is close to 35°C) of L. Plantarum, and the optimum time for LAB activity (> 12 hours), L. plantarum would produce more lactic acid, which will lead to a reduction in pH, increased TTA, and thus, higher acidity in the sourdough. Similar observations were also reported in previous studies [18, 22, 15].
Optimisation and validation process. RSM was used to develop a prediction model for optimising L. plantarum fermentation conditions for rice bran sourdough production. The independent and dependent variables were analysed to obtain a regression equation, that could predict response (pH and TTA) within the given range. The developed mathematical models for the rice bran sourdough are presented in Table 3. High values of R2 (the fit of the model to the experimental data) and Q2 (predictive power of the model) were obtained in the acidity model. The obtained R2 values (0.88) indicate that 88% of the variation can be explained by the fitted model (Table 3). Large Q2 values (> 0.9) imply that the model has a good predictive ability with a small prediction error. A significant linear effect of time and temperature was observed in pH values of the rice bran sourdoughs. The latter indicates that the influence of fermentation time on pH values was dependent on fermentation temperature. And both independent factors had quadratic effect on TTA values (Table 3). p-values for both models significantly affected the acidity levels with the p-value lower than 0.05, however, the p-value (0.000) for the reduced quadratic model of TTA was more significant than that of the pH linear model (p-value = 0.017). These results agreed with the results of the previous studies of L. plantarum fermented wheat sourdoughs [18, 33].
As shown in Fig. 2 and 3, the modelling of pH values and TTA produced different surface response plots for fermentation of rice bran sourdough at different fermentation time and temperature. In Fig.2 fermentation temperature and time displayed a linear effect on the pH, where the sourdough pH decreased with the increase of fermentation temperature and time. This was evident in the regression equation (Table 3), where fermentation temperature and time had a negative coefficient, which indicated a decrease in the response. In Fig. 3 fermentation temperature showed a significant effect on the TTA. The TTA of the sourdough increased with the increase of fermentation temperature, as evidenced in the regression equation (Table 3), where the multiplying effect of fermentation temperature (Te2) had a positive coefficient. This is also confirmed by the experimental analysis, where sourdough sample fermented at the highest temperature (35°C) had the highest TTA value (Fig. 1).
The levels of the independent variables generated a targeted range of pH (4.0 to 4.6) and TTA (14–23 ml of 0.1M NaOH/10g) for the rice bran sourdough (Fig. 1). The optimum pH for the sourdough was obtained in 19.5 hours at 34.8°C while, the optimum TTA value was obtained in 10.65 hours at 32.6°C. Based on the multiple optimisation of the fermentation of rice bran sourdough, the optimum pH value of 4.3 and TTA value of 19.5 ml of 0.1M NaOH were obtained, when the sourdough was fermented at 33°C for 12.5 hours.
A two-sample t-test was conducted to test the adequacy of the final reduced models by comparing the experimental values with predicted values. The data obtained showed that there were no significant differences (p > 0.05) between the experimental and predicted values (Table 4). This observation showed the agreement between the two values, and thus, the adequacy of the response surface equations fitted by RSM was verified.
The influence of fermentation parameters on total phenolic compounds. Since sourdough can improve phytochemicals in baked goods, we investigated the effect of fermentation conditions on the level of total phenolic compounds (TPC) in rice bran sourdough. The result is presented in Table 5. The highest amount of TPC (484.9 µg GAE/g) was detected in sourdough fermented at the high temperature (32.8°C) for a long time (17.95 hours). The least TPC (410.2 µg GAE/g) was found in rice bran sourdough that was fermented at a low temperature (22.2°C) for 8.05 hours. Although, the TPC values of the sourdoughs increased with the rise of fermentation temperature and time, there were not significant differences (p > 0.05) in the TPC of all the sourdoughs. This indicates that a high TPC value will be obtained at the optimum fermentation temperature (33°C) and time (12.5 hours). The trend of the TPC contents of the rice bran sourdoughs is in congruent with the previous findings on rye bran [5]. A higher TPC value obtained at a higher fermentation temperature and time could be due to the fermentation in liberating and synthesising bioactive compounds, such as phenolic compounds in cereal brans [5, 34, 35].
Volatile organic compounds (VOCs) of rice bran sourdough. The list of the volatile compounds detected in rice bran sourdough fermented at different temperature and time is presented in Table 6. It includes only those detected in more than one sample. Volatile analysis of the ten (10) rice bran sourdough samples (Table 2, A-J) showed the presence of 47 compounds. They were grouped into different chemical classes as esters (15), alcohols (7), acids (6), alkanes (5), pyridine (1), siloxane derivatives (3), aldehydes (2), phenol (1), furan (1), aromatic hydrocarbon (1), ketose (1), ketone (1), nitriles (1), and others (2). The VOCs from the rice bran sourdough could be generated from enzymatic and microbial processes during sourdough fermentation (e.g., acids, alcohol, esters, ketones, and aldehydes), lipid oxidation (e.g., aldehydes, and ketones), and Maillard and caramelisation reactions (e.g., furans, aldehydes, ketones, and pyridines), or from other compounds present in the flour [15].
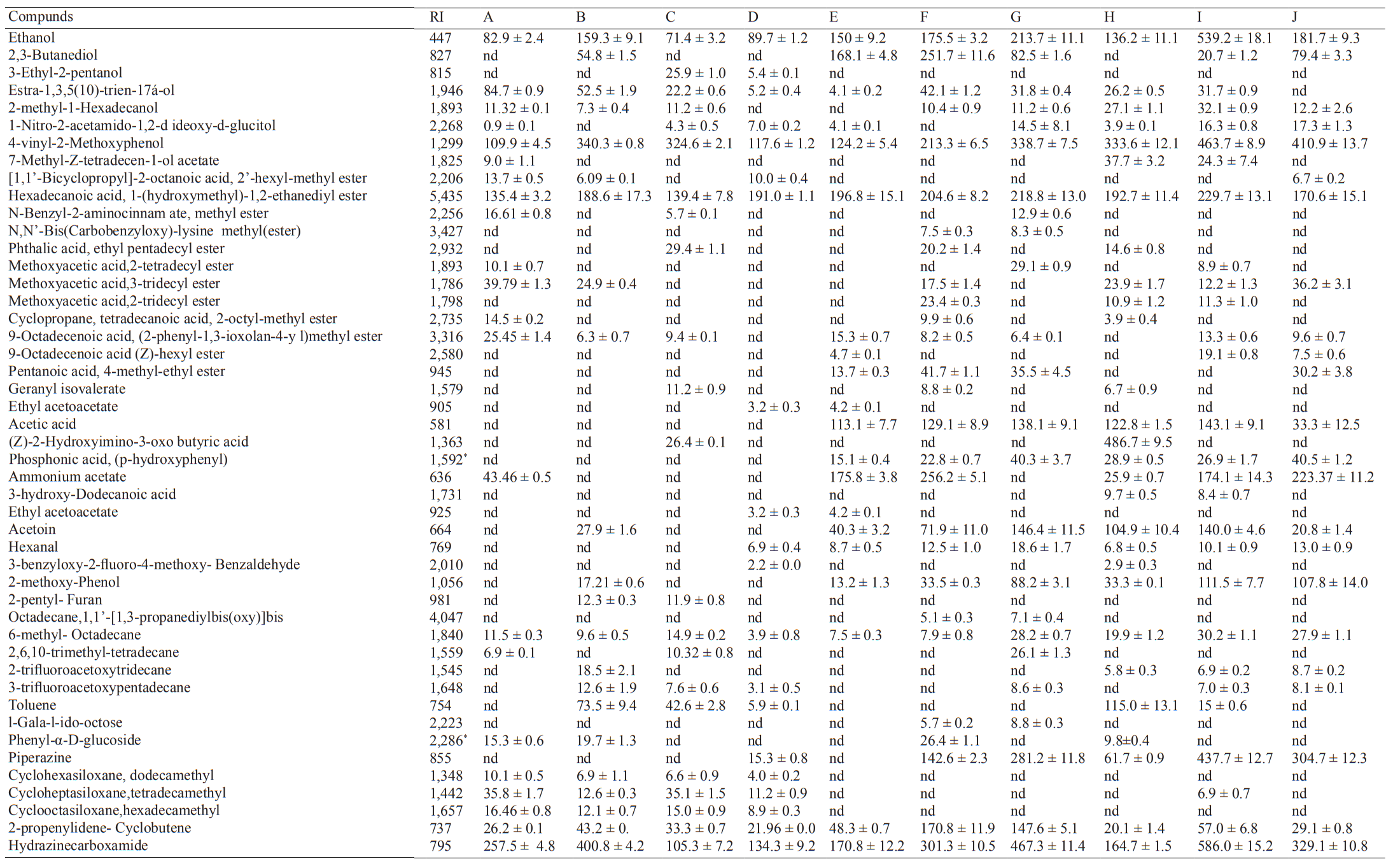
Note: Values are mean ± standard deviation (µg/kg) (n = 3). RI (Retention index): Identification by comparison with RI database. A–J: are as defined in Table 2. * indicates RI values not reported in the library for matching. nd: not detected
Rice bran sourdough fermented at a higher temperature (> 27°C) for a longer time (> 8 hours) produced the largest number of VOCs (Table 6). Furthermore, the amount of volatile compounds released from L. plantarum fermented rice bran sourdough (A-I) was more than the VOCs from naturally fermented rice bran sourdough (J). The most abundant alcohol among the VOCs was ethanol, a primary product of fermentation, followed by 2-Methoxy-4-vinylphenol. 2-Methoxy-4-vinylphenol (spicy) is an aromatic substance used as a flavouring agent [15, 36]. Ethanol production increased with the rise of fermentation temperature and time (Table 6). This could be because the longer fermentation time is enough for the microbial strain to degrade simple sugars in the sourdough into carbon-dioxide and ethanol [37]. Heterofermentative LAB has been reported to produce a high amount of ethanol and carbon-dioxide during rye sourdough fermentation [7], thus, L. plantarum (a facultatively heterofermentative LAB) [38] was probably responsible for increased ethanol and CO2 production during rice bran sourdough fermentation. Ethanol has been cited as a specific volatile compound in a wheat sourdough bread [15]. The highest amount of ethanol and 2-Methoxy-4-vinylphenol was detected in sourdough fermented at 35°C for 13h (sample I). This is an indication that rice bran sourdough can be a good alternative to wheat sourdough for bread production.
Estra-1,3,5(10)-trien-17á-ol, 2,3-Butanediol, 3-Ethyl-2-pentanol, 2-methyl-1-Hexadecanol, and 1-Nitro-2-acetamido-1,2-dideoxy-d-glucitol were other alcohols detected in the rice bran sourdoughs. Estra-1,3,5(10)-trien-17á-ol was present in all the L. plantarum fermented sourdough, but not found in the naturally fermented rice-bran sourdough (Table 6). This indicates that Estra-1,3,5(10)-trien-17á-ol is one of the main volatile compounds specific in L. plantarum fermented rice bran sourdoughs. This compound was also reported to be one of the main volatile compounds in moringa leave extract [40].
The presence of esters in sourdough and bread is an indicator of pleasant fruity aromas [39]. Esters in rice bran sourdough may be derived from products of free fatty acids reaction with some alcohols [41]. Hexadecanoic acid, 1-(hydroxymethyl)-1,2-ethanediyl ester, was the most abundant ester in rice bran sourdough, and its highest quantity was detected in the sample fermented at 35°C for 13 hours (I). Higher quantities of this ester were also found in sample F and G, which were fermented for more than 17 hours, indicating that the longer fermentation time favours the formation of the esters. According to the literature, the LAB fermentation requires more than 12 hours to produce a sufficient amount of volatiles [15]. The higher concentration of the esters in sample I, compared to sample F and G, could be, because sample I was fermented at 35°C for 13 hours, that was close to the optimum fermentation conditions (33°C for 12.5 hours) for rice bran sourdough production. Other esters found in the sourdough were 9-Octadecenoic acid, (2-phenyl-1,3-ioxolan-4-y l) methyl ester, Methoxyacetic acid, 3-tridecyl ester, and Pentanoic acid, 4-methylethyl ester. These esters were also detected at the relatively high amount in the sourdoughs. 9-Octadecenoic acid, (2-phenyl-1,3-ioxolan-4-y l) methyl ester, was also reported in buckwheat sprout [42].
Seven (7) esters were detected in two (2) samples (H and I) fermented at a high temperature for a short time (32.8°C, 8 hours; sample H) and a longer time (35°C, 13 hours; sample I). Although, rice bran sourdough fermented at the lowest temperature for a short time (20°C, 8 h; sample A) had the largest number of esters (8); the quantity of Hexadecanoic acid, 1-(hydroxymethyl)-1,2-ethanediyl ester, was the least in this sample. This indicates that high fermentation temperature and a long time favour the production of a large amount of esters in sourdough. However, there was variation in the type and quantity of esters present in all the rice bran sourdough samples.
A long fermentation time and a high temperature also favour the production of acids in the rice bran sourdoughs (Table 6). This observation is in conformity with the result of the sourdough acidity (Fig. 1), where sourdough sample fermented at 35°C for 13 hours had the highest acidity level. Sourdough acids have been reported to serve as a bio-preservative [39]. This is probably why L. plantarum fermented rice bran sourdough taftoon bread had a higher anti-mould activity than the doughs fermented by L. Casei, and L. acidophilus [13]. Rice bran sourdough fermented with L. plantarum at a higher temperature (> 32°C) for a longer time (> 12 h) (G and I) had the largest number of volatile acids. Thus, these sourdoughs can extend a bread shelf-life and improve its flavour [43, 44].
Lactic and acetic acids are important for the flavour and taste of sourdough bread [45]. Acetic acid is the most common volatile acids reported to be present in sourdoughs [46, 47]. This acid was detected in a large quantity (143 µg/kg) in rice bran sourdough fermented at 35°C for 13 hours (I), but not detected in the sourdough samples fermented at < 27°C for a short or long time. Even though LAB was used for sourdough fermentation, lactic acid was not detected in any of the rice bran sourdough samples.
Low levels of ketone were detected in the naturally fermented sourdough, compared to the L. plantarum fermented sourdough. The presence of ketone (acetoin) in the sourdough could be due to a long fermentation period. As shown in Table 6, the longer the fermentation time, the higher the amount of acetoin in the sourdoughs. Mild heating of flour for a long period under oxidising conditions has been reported to induce lipid peroxidation, consequently resulting in a lipid degradation to aldehydes and ketones [31]. The sourdough sample fermented at 32.8°C for 17:95 hours (G) had the higher acetoin value (146 µg/kg), than the sourdough fermented at 35°C for 13 hours (I). Acetoin was also reported to be present in a whole wheat sourdough [39] and chestnut-flour-based-sourdoughs [30]. Since acetoin is characterised with a butter, or popcorn like aroma [46, 47], the presence of acetoin in rice bran sourdough produced by fermentation of rice bran at a high temperature for a long time is an indicator, that the sourdough will add a special good flavour to bread, and retain bread freshness for a long time. The obtained results confirm the facts already known in the scientific literature [39].
Aldehydes are related to oxidative status of foods, characterised as off-odours in bread [46, 47]. The presence of aldehydes (hexanal and 3-benzyloxy-2-fluoro-4-methoxy- Benzaldehyde) in the rice bran sourdoughs could be due to the activities of lipid hydrolysing enzymes, such as lipases, and peroxidases present in bran [48]. The detection of linear chain aldehyde (hexanal) could be due to degradative oxidation of unsaturated fatty acids, such as oleic, linoleic, and linolenic acids [31], in which rice bran is rich [1]. Although the concentration of aldehydes in all the samples was low, a relatively high concentration of hexanal in samples F, G, I, and J, compared to other samples, could be due to the susceptibility of the samples to oxidative rancidity. Non detection of hexanal in samples A, B, and C could probably be explained by the activities of lipase, reduced at the temperature of 20–22.2°C. The storage of bran at a low temperature has been reported to hinder the activity of lipases [49].
Phenol (2-methoxy-Phenol) is one of the compounds responsible for the aroma of buckwheat [50]. It was present in all the sourdoughs, except those fermented at a low temperature (20–27.5°C) for a short and a long time (6–13 h) (A, C, and D). 2-methoxy-Phenol is formed during the thermal degradation of lignin under the pyrolysis condition or thermal degradation of barks [51]. This cannot be the case of this study, since the rice bran, or sourdough was not subjected to a high temperature. However, the contamination of rice bran during drying or dehusking of rice paddy could be responsible for the presence of the compound in the sourdoughs.
Furan (2-pentyl- Furan), characterised with a fruity odour [15], was detected only in the samples fermented at a low temperature for a long time (B and C). Although formation of heterocyclic compounds, such as furans were attributed to the thermal degradation and rearrangement of carbohydrate through Maillard reaction [31, 52], this may not be the case of this study. Since the headspace incubation temperature was 60°C, and Maillard reaction products are produced at a temperature between 80 to 120°C [42, 53]. The presence of 2-pentyl-Furan in rye sourdough and in wheat bread without sourdough has been reported [7, 47, 54]. 6-methyl-Octadecane was the only alkane found in all the sourdough samples, even though in a varying concentration. All other alkanes vary from one sample to another in terms of their detection and quantity. Aromatic hydrocarbon (toluene), ketose (l-Gala-l-ido-octose), and nitrile (Phenyl-α-D-glucoside) were not detected in a naturally fermented sample, but were detected at low concentrations in less than six (6) L. plantarum fermented sourdoughs. Nitrile was found in low amount in samples A, B, F, and H, but not detected in other sourdough samples, whereas pyridine (piperazine) was not found in samples A, B, C, and E, but was detected in other sourdoughs. Most of the siloxane derivatives found in the sourdough were detected in the samples fermented at a low temperature (A, B, C, and D), except for Cycloheptasiloxanetetradecamethyl, which was found in a low concentration in sample I. The 3 siloxane derivatives found in the rice bran sourdoughs are among the 4 main volatile compounds found in jasmine flower [55]. l-Gala-l-ido-octose was found only in two samples (F and G), while 2-propenylidene-Cyclobutene was detected in all the sourdough samples, except sample B. Hydrazinecarboxamide was found in a high concentration in all the sourdoughs.
Generally, it was observed that rice bran sourdough samples fermentation at the higher temperature and time had the higher concentration of 4-vinyl-2-Methoxyphenol, ethanol, 2-methoxy- Phenol, acetoin, and piperazine. However, an opposite trend was observed in the case of siloxane derivatives and aldehydes, where there was low or no detection of the compounds, as fermentation temperature and time increased (Table 6). Meanwhile, fluctuating levels of alkanes, aromatic hydrocarbon, nitrile, 2-propenylidene-cyclobutene, esters, and alcohols (apart from 4-vinyl-2-Methoxyphenol, and ethanol) were detected in the rice bran sourdoughs. These observations were in agreement with the findings of [39], who reported the reduction in percent peak area of aldehydes and fluctuating levels of alcohols, esters, and acids in a whole wheat sourdough fermented for a long time.
According to the results of this study, sourdough fermented at 35°C for 13 hours (sample I) contained the largest amount (27) of aroma compounds, compared to other L. plantarum fermented sourdough, and eight (8) compounds more than the naturally fermented sourdough (sample J). Although sample I and sample J were fermented at the same temperature and time (35°C for 13 hours), the following VOCs were found in sample I: estra-1,3,5(10)-trien-17á-ol, 2-methyl-1-Hexadecanol, 3-hydroxy-dodecanoic acid, 7-methyl-z-tetradecen-1-olacetate, methoxyaceticacid 2-tetradecyl ester, methoxyacetic acid 2-tridecyl ester, cycloheptasiloxanetetradecamethyl, and toluene. However, these VOCs were not detected in the naturally fermented sourdough (J).
Acetic acid and ethanol are among the most cited volatile organic compounds found in bread crust [47], crumb [46], and wheat bread without sourdough [15] and with sourdough [39]. Sample I had the highest concentration of acetic acids (143 µg/kg), ethanol (539 µg/kg), 2-Methoxy-4-vinylphenol (463 µg/kg), Hexadecanoic acid, 1-(hydroxymethyl)-1,2-ethanediyl ester (230 µg/kg), and 2-methoxy-Phenol (112 µg/kg) compared to all other sourdough samples. Sample I is also high in the aroma compound acetoin (140 µg/kg). The higher VOCs in sample I could be due to the fermentation conditions (33°C, 13 h), which are close to the optimum fermentation conditions for rice bran sourdough production.These characteristics of sample I indicate that the sourdough will produce bread with high consumer acceptability, extended freshness, and shelf-life.
ВЫВОДЫ
The results of this study demonstrate that fermentation temperature and time have a significant effect on the quality parameters of rice bran sourdough. Acidity, total phenolic, and volatile compounds of rice bran sourdough increased, as fermentation temperature and time increased. The optimum fermentation conditions for L. plantarum fermented rice bran sourdough were achieved at 33°C for 12.5 hours. These conditions resulted in pH value of 4.3 and TTA value of 19.5 ml of 0.1M NaOH, which was not significantly different from the RSM predicted pH (4.2), and TTA (19.1ml of 0.1M NaOH) values. Fermentation temperature and time do not have a significant effect on the total phenolic content of the sourdough. Forty-seven (47) VOCs were detected in the rice bran sourdoughs. As fermentation temperature and time increased, the concentration of ethanol, phenols, ketone, pyridine, and acids increased in the rice bran sourdoughs, while aldehydes and siloxane derivatives levels decreased. The major volatile compounds in the sourdough were acetic acids, ethanol, 2-Methoxy-4-vinylphenol, Hexadecanoic acid, 1-(hydroxymethyl)-1,2-ethanediyl ester, acetoin, and 2-methoxy-Phenol. There were striking differences between the volatile composition of L. plantarum fermented rice bran sourdoughs and the naturally fermented sourdough. Differences also existed in the sourdough fermented with L. plantarum at a high temperature and time and those fermented at a low temperature and time. Former had the higher concentration of volatile aroma compounds than the latter. The information provided in this study can be used in bakery industry for producing quality shelf-stable rice bran sourdough bread with attractive aromas.
БЛАГОДАРНОСТИ
The research was supported by Universiti Putra Malaysia and Padiberas NasionalBerhad (Vot. 6360600). The author I.F. Bolarinwa is grateful to Universiti Putra Malaysia (UPM) and the World Academy of Science (TWAS) for postdoctoral fellowship award and to Ladoke Akintola University of Technology, Nigeria.
СПИСОК ЛИТЕРАТУРЫ
- Chinma C.E., Ramakrishnan Y., Ilowefah M., Hanis-Syazwani M., and Muhammad K. Properties of cereal brans: a review. Cereal Chemistry, 2015, vol. 92, pp. 1–7. DOI: https://doi.org/10.1094/CCHEM-10-13-0221-RW.
- Katina K., Salmenkallio-Marttila M., Partanen R., Forssell P., and Autio K. Effects of sourdough and enzymes on staling of high-fibre wheat bread. LWT – Food Science Technology, 2006, vol. 39, no. 5, pp. 479–491. DOI: https:// doi.org/10.1016/j.lwt.2005.03.013.
- Coda R., Katina K., and Rizzello C.G. Bran bioprocessing for enhanced functional properties. Current Opinion in Food Science, 2015, vol. 1, no. 1, pp. 50–55. DOI: https://doi.org/10.1016/j.cofs.2014.11.007.
- Sanz Penella J.M., Collar C., and Haros M. Effect of wheat bran and enzyme addition on dough functional perfor- mance and phytic acid levels in bread. Journal of Cereal Science, 2008, vol. 48, no. 3, pp. 715–721. DOI: https://doi. org/10.1016/j.jcs.2008.03.006.
- Katina K., Laitila A., Juvonen R., et al. Bran fermentation as a means to enhance technological properties and bioac- tivity of rye. Food Microbiology, 2007, vol. 24, no. 2, pp. 175–186. DOI: https://doi.org/10.1016/j.fm.2006.07.012.
- Coda R., Kärki I., Nordlund E., et al. Influence of particle size on bioprocess induced changes on technological func- tionality of wheat bran. Food Microbiology, 2014, vol. 37, pp. 69–77. DOI: https://doi.org/10.1016/j.fm.2013.05.011.
- Kaseleht K., Paalme T., Mihhalevski A., and Sarand I. Analysis of volatile compounds produced by different species of lactobacilli in rye sourdough using multiple headspace extraction. International Journal of Food ScienceTechnolo- gy, 2011, vol. 46, no. 9, pp. 1940–1946. DOI: https://doi.org/10.1111/j.1365-2621.2011.02705.x.
- Gobbetti M., Rizzello C.G., Di Cagno R., and De Angelis M. How the sourdough may affect the functional features of leavened baked goods. Food Microbiology, 2014, vol. 37, pp. 30–40. DOI: https://doi.org/10.1016/j.fm.2013.04.012.
- Corsetti A., Gobbetti M., De Marco B., et al. Combined Effect of Sourdough Lactic Acid Bacteria and Additives on Bread Firmness and Staling. Journal of Agricultural and Food Chemistry, 2000, vol. 48, no. 7, pp. 3044–3051. DOI: https://doi.org/10.1021/jf990853e.
- Crowley P., Schober T., Clarke C., and Arendt E. The effect of storage period on textural and crumb grain charac- teristics of sourdough wheat bread. European Food Research and Technology, 2002, vol. 214, no. 6, pp. 489–496. DOI: https://doi.org/10.1007/s00217-002-0500-7.
- Moore M.M., Bello F.D., and Arendt E.K. Sourdough fermented by Lactobacillus plantarum FST 1.7 improves the quality and shelf life of gluten-free bread. European Food Research Technology, 2008, vol. 226, no. 6, pp. 1309–1316. DOI: https://doi.org/10.1007/s00217-007-0659-z.
- Torrieri E., Pepe O., Ventorino V., Masi P., and Cavella S. Effect of sourdough at different concentrations on qual- ity and shelf life of bread. LWT – Food Science Technology, 2014, vol. 56, no. 2, pp. 508–516. DOI: https://doi. org/10.1016/j.lwt.2013.12.005.
- Torkamani M.G., Razavi S.H., and Gharibzahedi S.M.T. Critical quality of Iranian “Taftoon” breads as affected by the addition of rice bran sourdough with different lactobacilli. Quality Assurance Safety Crops Foods, 2015, vol. 7, no. 3, pp. 305–311. DOI: https://doi.org/10.3920/QAS2013.0375.
- Farahmand E., Razavi S.H., Yarmand M.S., and Morovatpour M. Development of Iranian rice-bran sourdough breads: physicochemical, microbiological and sensorial characteristics during storage period. Quality Assurance Safety Crops Foods, 2015, vol. 7, no. 3, pp. 295–303. DOI: https://doi.org/10.3920/QAS2013.0334.
- Petel C., Onno B., and Prost C. Sourdough volatile compounds and their contribution to bread: A review. Trends Food Science and Technology, 2017, vol. 59, pp. 105–123. DOI: https://doi.org/10.1016/j.tifs.2016.10.015.
- Meuser F. and Valentin M. Fermented doughs in bread production. In: Hui Y.H., Meunier-Goddik L., Josephsen J., Nip W.-K., Stanfield P.S.(eds) Handbook of Food and Beverage Fermentation Technology. New York: CRC Press Publ., 2004. 757–782 p.
- Brandt M.J., Hammes W.P., and Ganzle M.G. Effects of process parameters on growth and metabolism of Lactobacil- lus sanfranciscencis and Candida humilis during rye sourdough fermentation. European Food Research Technology, 2004, vol. 218, no. 4, pp. 333–338. DOI: https://doi.org/10.1007/s00217-003-0867-0.
- Katina K., Poutanen K., and Autio K. Influence and Interactions of Processing Conditions and Starter Culture on Formation of Acids, Volatile Compounds, and Amino Acids in Wheat Sourdoughs. Cereal Chemistry, 2004, vol. 81, no. 5, pp. 598–610. DOI: https://doi.org/10.1094/CCHEM.2004.81.5.598.
- Bezerraa M.A., Santelli R.E., Oliveiraa E.P., Villar L.S., and Escaleira L.A. Response surface methodology (RSM) as a tool for optimization in analytical chemistry. Talanta, 2008, vol. 76, no. 5, pp. 965–977. DOI: https://doi. org/10.1016/j.talanta.2008.05.019.
- Chinma C. E., Ilowefah M., and Muhammad K. Optimization of Rice Bran Fermentation Conditions Enhanced by Baker’s Yeast for Extraction of Protein Concentrate. Nigeria Food Journal, 2014, vol. 32, no. 1, pp. 126–132. DOI: https://doi.org/10.1016/S0189-7241(15)30105-3.
- Hanis-Syazwani M., Bolarinwa I.F., Lasekan O., and Muhammad K. Influence of starter culture on the physicochem- ical properties of rice bran sourdough and physical quality of sourdough bread. Food Research, 2018, vol. 2, no. 4, pp. 340–349. DOI: https://doi.org/10.26656/fr.2017.2(4).045.
- Hansen A. and Schieberle P. Generation of aroma compounds during sourdough fermentation: applied and fundamen- tal aspects. Trends in Food Science and Technology, 2005, vol. 16, no. 1–3, pp. 85–94. DOI: https://doi.org/10.1016/j. tifs.2004.03.007.
- Sukhonthara S., Theerakulkait C., and Miyazawa M. Characterization of Volatile Aroma Compounds from Red andBlack Rice Bran. Journal of Oleo Science, 2009, vol. 58, no.3, pp. 155–161. DOI: https://doi.org/10.5650/jos.58.155.
- Joglekar A.M. and May A.T. Product excellence through design of experiments. Cereal Food World, 1987, vol. 32, pp. 857–868.
- Gül H., Özçelik S., Sa˘gdıç O., and Certel M. Sourdough bread production with lactobacilli and S. cerevisiae isolated from sourdoughs. Process Biochemistry, 2005, vol. 40, no. 2, pp. 691–697. DOI: https://doi.org/10.1016/j.proc- bio.2004.01.044.
- Hansen A. and Hansen B. Influence of Wheat Flour Type on the Production of Flavour Compounds in Wheat Sour- doughs. Cereal Science, 1994, vol. 19, no. 2, pp. 185–190. DOI: https://doi.org/10.1006/jcrs.1994.1025.
- Brummer J.M. and Lorenz K. European developments in wheat sourdoughs. Cereal Foods World, 1991, vol. 36, pp. 310–314.
- Thondre P.S., Ryan L., and Henry C.J.K. Barley ß-glucan extracts as rich sources of polyphenols and antioxidants.Food Chemistry, 2011, vol. 126, no. 1, pp. 72–77. DOI: https://doi.org/10.1016/j.foodchem.2010.10.074.
- Nevskayaa E.V., Borodulinb D.M., Potekhac V.L., et al. Development of integrated technology and assortment of long-life rye-wheat bakery products. Foods and Raw Materials, 2018, vol. 6, no. 1, pp. 99–109. DOI: https://doi. org/10.21603/2308-4057-2018-1-99-109.
- Aponte M., Boscaino F., Sorrentino A., et al. Volatile compounds and bacterial community dynamics of chestnut- flour-based sourdoughs. Food Chemistry, 2013, vol. 141, no. 3, pp. 2394–2404. DOI: https://doi.org/10.1016/j. foodchem.2013.05.052.
- Cirlini M., Dall’Asta C., Silvanini A., et al. Volatile finger printing of chestnut flours from traditional Emilia Romagna (Italy) cultivars. Food Chemistry, 2012, vol. 134, no. 2, pp. 662–668. DOI: https://doi.org/10.1016/j.food- chem.2012.02.151.
- Van den Dool H. and Kratz P.D. A generalization of the reaction of the retention index system including linear tem- perature programmed gas–liquid partition chromatography. Journal of Chromatography A, 1963, vol. 11, pp. 463–471.
- Flander L., Suortti T., Katina K., and Poutanen K. Effects of wheat sourdough process on the quality of mixed oat- wheat bread. LWT – Food Science Technology, 2011, vol. 44, no. 3, pp. 656–664. DOI: https://doi.org/10.1016/j. lwt.2010.11.007.
- Katina K., Liukkonen K-H., Kaukovirta-Norja A., et al. Fermentation-induced changes in the nutritional value of na- tive and germinated rye. Journal of Cereal Science, 2007, vol. 46, no. 3, pp. 348–355. DOI: https://doi.org/10.1016/j. jcs.2007.07.006.
- Loponen J., Mikola M., Katina K., Sontag-Strohm T., and Salovaara H. Degradation of HMW Glutenins During Wheat Sourdough Fermentations. Cereal Chemistry, 2004, vol. 81, no. 1, pp. 87–90. DOI: https://doi.org/10.1094/ CCHEM.2004.81.1.87.
- FAO/WHO (2001). Summary of evaluations performed by the Joint FAO/WHO Expert Committee on food additives (2-methoxy-4-vinylphenol). Available at: http://www.inchem.org/documents/jecfa/jeceval/jec_1395.htm. (accessed 12 September 2018).
- De Vuyst L., Harth H., Kerrebroeck S.V., and Leroy F. Yeast diversity of sourdoughs and associated metabolic prop- erties and functionalities. International Journal of Food Microbiology, 2016, vol. 239, pp. 26–34. DOI: https://doi. org/10.1016/j.ijfoodmicro.2016.07.018.
- Corsetti A. and Settanni L. Lactobacilli in sourdough fermentation. Food Research International, 2007, vol. 40, no. 5, pp. 539–558. DOI: https://doi.org/10.1016/j.foodres.2006.11.001.
- Pizarro F. and Franco F. Volatile Organic Compounds at Early Stages of Sourdough Preparation Via Static Headspace and GC/MS Analysis. Current Research in Nutrition and Food Science, 2017, vol. 5, pp. 89–99. DOI: https://doi. org/10.12944/CRNFSJ.5.2.05.
- Shokery E.S., El-Ziney M.G.,Yossef A.H., and Mashaly R.I. Effect of Green Tea and Moringa Leave Extracts Fortifi- cation on the Physicochemical, Rheological, Sensory and Antioxidant Properties of Set-Type Yoghurt. Advance Dairy Research, 2017, vol. 5, no. 2, pp. 1–10. DOI: https://doi.org/10.4172/2329-888X.1000179.
- Özgül-Yücel S. and Türkay S. Variables affecting the yields of methyl esters derived from in situ esterification of rice bran oil. Journal of American Oil Chemistry Society, 2002, vol. 79, no. 6, pp. 611–614. DOI: https://doi.org/10.1007/ s11746-002-0531-5.
- Kim J. and Lee Y. Study of Maillard reaction products derived from aqueous model systems with different peptide chain lengths. Food Chemistry, 2009, vol. 116, no. 4, pp. 846–853. DOI: https://doi.org/10.1016/j.foodchem.2009.03.033.
- Hansen A. and Hansen B. Flavour of sourdough wheat bread crumb. Journal of Food Control Research, 1996, vol. 202, no. 3, pp. 244–249. DOI: https://doi.org/10.1007/BF01263548.
- Birch A.N., Petersen M.A., and Hansen Å.S. REVIEW: Aroma of Wheat Bread Crumb. Cereal Chemistry, 2014, vol. 91, no. 2, pp. 105–114. DOI: https://doi.org/10.1094/CCHEM-06-13-0121-RW.
- Pico J., Bernal J., and Gómez M. Wheat bread aroma compounds in crumb and crust: A review. Food Research Inter- national, 2015, vol. 75, pp. 200–215. DOI: https://doi.org/10.1016/j.foodres.2015.05.051.
- Saunders R.M. The properties of rice bran as a foodstuff. Cereal Foods World, 1990, vol. 35, no. 7, pp. 632–636.
- Prakash J. Rice bran proteins: Properties and food uses. Critical Review of Food Science and Nutrition, 1996, vol. 36, no. 6, pp. 537–552. DOI: https://doi.org/10.1080/10408399609527738.
- Janes D., Kantar D., Kreft S., and Prosen H. Identification of buckwheat (Fagopyrum esculentum Moench) aroma compounds with GC-MS. Food Chemistry, 2008, vol. 112, no. 1, pp. 120–24. DOI: https://doi.org/10.1016/j.food- chem.2008.05.048.
- Gruber M.A. The flavor contributions of kilned and roasted products to finished beer styles. Technical Quarterly, 2010, vol. 38, pp. 227–233.
- Matsakidou A., Blekas G., and Paraskevopoulou A. Aroma and physical characteristics of cakes prepared by replacing margarine with extra virgin olive oil. LWT – Food Science Technology, 2010, vol. 43, no. 6, pp. 949–957. DOI: https:// doi.org/10.1016/j.lwt.2010.02.002.
- Martins S.I. and Boekel M.A. A kinetic model for the glucose/glycine Maillard reaction pathways. Food Chemistry, 2005, vol. 90, no. 1–2, pp. 257–269. DOI: https://doi.org/10.1016/j.foodchem.2004.04.006.
- Raffo A., Carcea M., Castagna C., and Magri A. Improvement of a headspace solid Phase microextraction-gas/chroma- tography mass spectrometry method for the analysis of wheat bread volatile compounds. Journal of Chromatography A, 2015, vol. 1406, pp. 266–278. DOI: https://doi.org/10.1016/j.chroma.2015.06.009.
- Ray H., Majumdar S., Biswas S.P., et al. Characterization of the Volatile Aroma Compounds from the Concrete and Jasmine Flowers Grown in India. Chemical Engineering Transactions, 2014, vol. 40, pp. 265–270. DOI: https://doi. org/10.3303/CET1440045.